By Carl Johannink and Paul Roggemans
Weather provided very good observing conditions around the traditional Lyrid maximum. 1929 orbits were collected in 27 (partly) clear nights, of which 203 could be identified as members of the Lyrid stream. The radiant drift in R.A. was +0.87 ± 0.08 degrees per day. De radiant drift in declination was -0.10 ± 0.11 degrees per day, based upon the 106 Lyrids obtained in the period between April 17/18 and April 23/24 fulfilling the D-criterion < 0.04.
1 Introduction
The month of April was characterized by an abrupt transition of rather cold to mainly very warm weather. The change occurred about the 10th of April. It was the start of an overall sunny period which persisted until almost the end of the month. The timing was perfect with mostly clear nights around the traditional Lyrid maximum.
2 The data
The month of April counted only three nights without any double station meteors. Beyond April 14–15, also the two last nights of the month had zero coincidences. Especially the nights in the period of 16 until 23 April had very clear nights. Hence, it is no surprise that most double station meteors were obtained during the nights near the Lyrid maximum.
In total 1929 orbits were registered by our network, a new record for this month in spring. Figure 1 displays the radiant positions of all registered orbits. The strong concentration around α = 270° and δ = +30° is indeed due to the Lyrids.
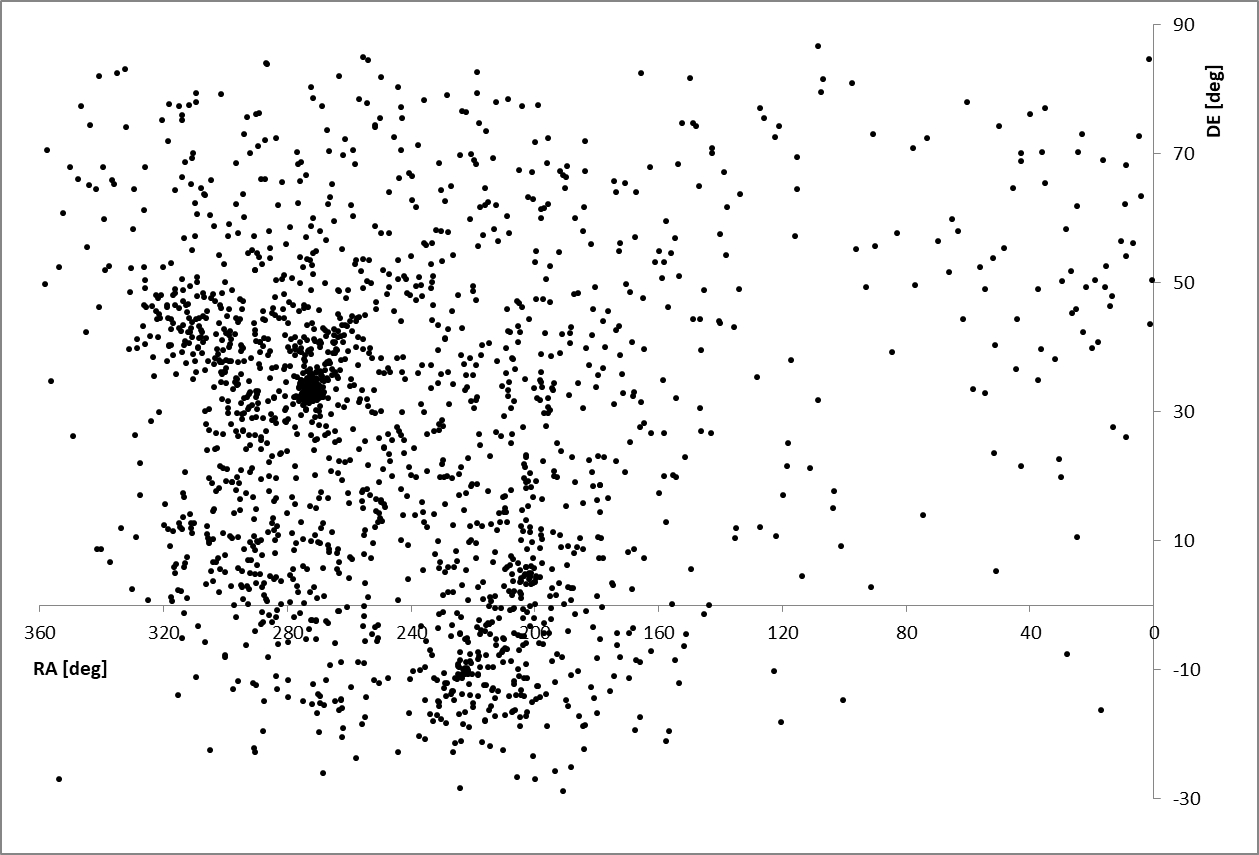
Figure 1 – Radiant positions of the 1929 orbits collected by CAMS BeNeLux in April 2018.
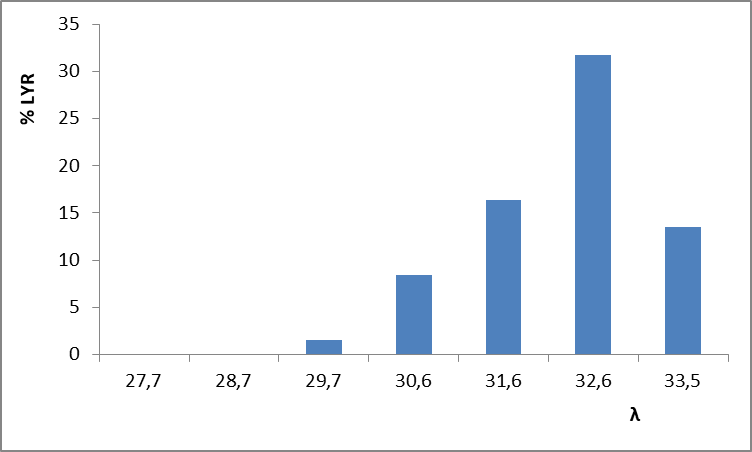
Figure 2 – Percentage of Lyrids relative to the other meteors during the nights 17-18 until 23-24 April 2018. (Source: CAMS BeNeLux data).
To distinguish the Lyrid orbits from the other meteors, the D-criterion of Drummond has been applied (Drummond, 1981). A total of 106 orbits were found which fulfilled this Drummond criterion DD < 0.04. Figure 2 shows for each of the nights of 17–18 April until 23–24 April the percentage of Lyrids of the dataset relative to the number of other meteors in the same night. We see a nice activity profile which is in good agreement with the known profile with a maximum activity around solar longitude 32°.
The number of Lyrids is large enough to derive the radiant drift around the maximum for the period of 17 until 23 April (λʘ ~ 29.5° – 33.6°). In Figure 3 we see a radiant drift in Right Ascension of 0.87 ± 0.08°/λʘ. Figure 4 shows the radiant drift in declination with –0.10 ±0.11°/λʘ.
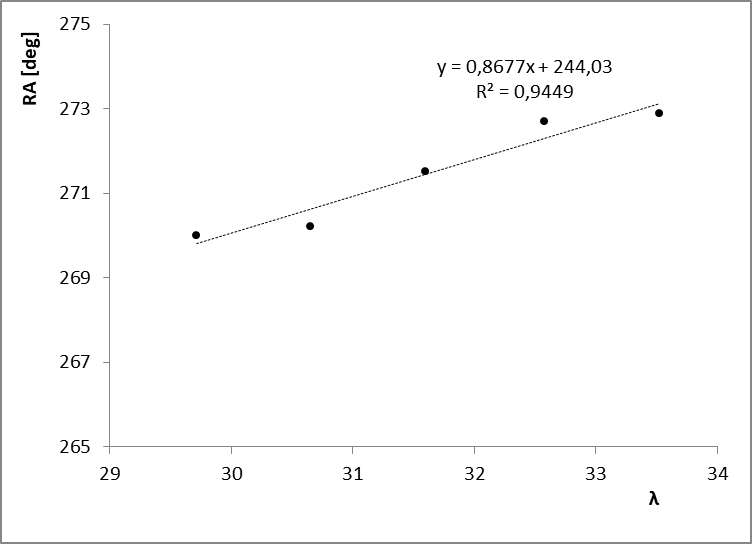
Figure 3 – Radiant drift in Right Ascension for the Lyrids 2018. (Source: CAMS BeNeLux data).
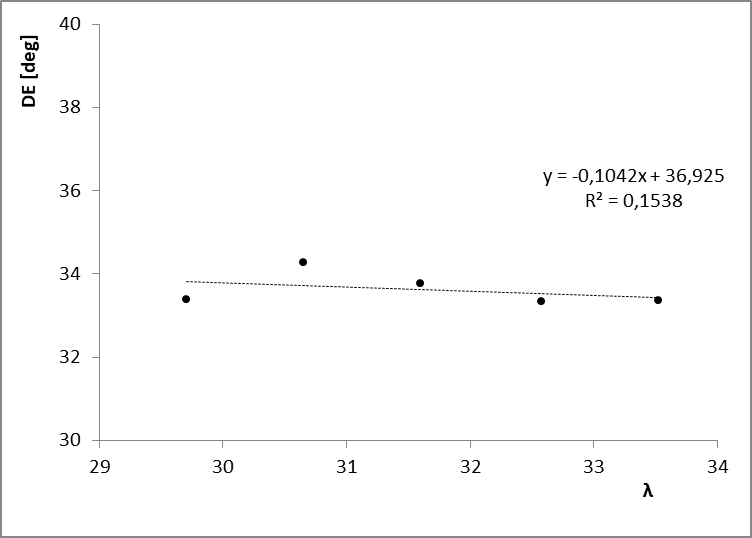
Figure 4 – Radiant drift in declination for the Lyrids 2018. (Source: CAMS BeNeLux data).
The standard deviation with these values has been derived using a matrix in Excel which does not only calculate the best linear fit, but also provides the standard deviation on the slope (http://pages.mtu.edu/~fmorriso/cm3215/UncertaintySlopeInterceptOfLeastSquaresFit.pdf) (Bouma and Doom, 2018).
Jenniskens et al. (2016) gives a drift for the Right Ascension and declination of respectively +0.66° and +0.02°. Our results are still within the error margin compared to the reference for the drift in declination. This is not the case for the drift in Right Ascension as our value is a bit larger than the value given by Jenniskens et al. (2016).
In order to check our data, we compared with some other public available datasets. We used the datasets of Edmond and SonotaCo. We selected the following data from these datasets:
- Solar longitude between 19° and 44°;
- Right Ascension of the radiant between 257° and 287°;
- Declination of the radiant between +23° and +44°;
Geocentric velocity between 41.7 and 51.7 km/s.
3533 orbits fulfilled the above-mentioned criteria. The median values for the orbital elements q, e, i, ω and Ω of this selection are representative for the Lyrids and have been used as parent orbit to compute the D-criteria. The median values of the orbits with DD < 0.04 were used as final reference orbit for the Lyrid to re-compute the D-criteria. For the 1750 Lyrid orbits with a value of DD < 0.04 the median values for the solar longitude, geocentric radiant position, geocentric velocity and orbital elements were computed. The results are listed in Table 1.
Using only orbits DD < 0.04 in the time lapse of 28.2° until 35.9° in solar longitude for the Edmond and SonotaCo data we find a drift of +1.04±0.03° in Right Ascension and –0.21±0.02° in declination. These values differ a lot from those given in literature (Jenniskens et al., 2016).
Table 1 – Median values for the radiant positions, vg and orbital elements of the Lyrids obtained from data of CAMS BeNeLux (2018), Edmond/SonotaCo and Jenniskens et al. (2018).
BeNeLux (2018) | EDMONd / SonotaCo | Jenniskens et al. (2018) | |
λʘ | 32.5° | 32.3° | 31.7° |
αg | 272.3° | 272.1° | 272.1° |
Δα | 0.87±0.08° | 1.04±0.03° | +0.66° |
δg | +33.4° | +33.4° | +33.6° |
Δδ | -0.10±0.11° | -0.21±0.02° | +0.02° |
vg | 46.8 km/s | 46.5 km/s | 46.7 km/s |
a | 29.9 AU | 14.9 AU | 25.6 AU |
q | 0.9208 AU | 0.919 AU | 0.923 AU |
e | 0.969 | 0.943 | 0.964 |
ω | 213.9° | 214.5° | 213.6° |
Ω | 32.5° | 32.3° | 31.7° |
i | 79.7° | 79.3° | 79.3° |
N | 106 | 1750 | 1249 |
3 Conclusion
The Lyrids could be very well observed this year. The radiant drift could be derived from the available data. For the declination our value is still within the margin of the literature value. For data from Edmond and SonotaCo we find a slight but clearly negative drift for the declination.
For the Right Ascension we find a drift of +0.87±0.08° from our data, a slightly larger value than the value in literature. For the Edmond/SonotaCo data the resulting drift in Right Ascension is even larger with 1 degree per day.
Acknowledgment
The authors thank Reinder Bouma for his critical reading of the article and his advices. Also thanks to Claude Doom for his help with the calculations in Excel.
References
Bouma R. and Doom C. (2018). Private communications.
Drummond J. D. (1981). “A test of comet and meteor shower associations”. Icarus, 45, 545–553.
Jenniskens P., Gural P. S., Grigsby B., Dynneson L., Koop M. and Holman D. (2011). “CAMS: Cameras for Allsky Meteor Surveillance to validate minor meteor showers”. Icarus, 216, 40–61.
Jenniskens P., Nénon Q., Albers J., Gural P. S., Haberman B., Holman D., Morales R., Grigsby B. J., Samuels D. and Johannink C. (2016). “The established meteor showers as observed by CAMS”. Icarus, 266, 331–354.
Jenniskens P., Baggaley J., Crumpton I., Aldous P., Pokorny P., Janches D., Gural P. S., Samuels D., Albers J., Howell A., Johannink C., Breukers M., Odeh M., Moskovitz N., Collison J. and Ganjuag S. (2018). “A survey of southern hemisphere meteor showers”. Planetary Space Science, 154, 21–29.