Jakub Koukal¹
¹ Valašské Meziříčí Observatory, Vsetínská 78, 75701 Valašské Meziříčí, Czech Republic
j.koukal@post.cz
Meteor astronomy is a very young branch of astronomy, its development began only 200 years ago. Even in the 18th century, there was a presumption that the meteors are present in the Earth’s atmosphere, that their origin is not extraterrestrial. There are more than 2500 years old observations of meteor showers (Lyrids meteor shower in 687 BC or Perseids in 36 AD), but science has not been interesting until the end of the 18th century. The great boom of meteoric astronomy occurred in the mid-20th century with the advent of new observation methods – radar and photographic observations. However, the emergence of the use of video technology for meteor studies has meant an unprecedented boom of this branch of astronomy since the 1990s, with lots of new meteor data and a huge number of newly discovered meteor showers. And this fact is problematic and shows that the methods used so far will have to be modified to reflect all aspects of new trends in the field of meteoric astronomy.
History of meteor showers research
The first pioneer of the true nature of the meteors was Ernst F.F. Chladni, who published in 1794 the book “Über den Ursprung der von Pallas gefundenen und anrer ihr änlicher Eisenmassen und über einige damit in Verbindung stehende Naturerscheinungen”, which first dealt with the origin of meteoroids and demolished the two myths of the origin of these bodies: (1) fragments of stone and iron bodies fall from the sky and (2) there are no small bodies in the space behind the orbit of the Moon. In 1807 Atanasije Stojković published the first monograph on meteors. Nevertheless, the monograph “O воздушных камнях и их произхождений” did not deal with the astronomical aspect of this phenomenon and, moreover, was a unique act in the field of nascent meteoric astronomy. In 1833, during the great meteor storm of Leonids meteor shower (on the night of November 12-13), Denison Olmsted noticed that the meteors were flying out of the sky from one place. He was the first who was able to observe the radiant of the meteor shower, and rightly concluded that this was a prospective phenomenon.
In 1866, Giovanni Virginio Schiaparelli carried out a Perseids observation analysis from 1864-1866 and first calculated a reliable meteoroid orbit in the Solar System. He also found the connection between Perseids and newly discovered comet Swift-Tuttle-Simons 1862 III. The same was done in co-operation with Urbain Le Verriere and Theodore von Oppolzer in the case of the meteor shower Leonids in 1866 when a connection between the meteor storm of Leonids and the comet Tempel-Tuttle 1866 I was established. Thanks to these discoveries, the idea that the meteors are the result of the comets collapse and that they are not different bodies was promoted. This view was confirmed after the collapse of the comet 3D/Biela in 1852 and the subsequent meteor storm of Andromedids meteor shower in 1872 and 1885. Already at the turn of the 19th and 20th centuries, several weaker meteor showers were discovered, due to the lack of observations for their precise determination, there were long disputes about their existence.
The decline of meteoric astronomy in the first half of the 20th century was halted in the 1940s when radars and photo chambers began to be used in meteoric astronomy to detect accurate meteor paths in the atmosphere. Until the onset of video technology, the IAU MDC Photographic Meteor Orbits Database (Lindblad et al., 2003), along with the catalog of radio observation orbits, was the only source for discovering and analyzing new meteor showers. The first recorded photographic orbit in the IAU MDCPhotographic Meteor Orbits Database dates back to 1936, sporadically discovered orbits began to increase rapidly in the 1950s in the context of the development of photographic networks, and currently includes the IAU MDC Photographic Meteor Orbits Database 4873 multi-station orbits. The European bolid network, which was started by Zdeněk Ceplecha in the 1950s and was fully operational in 1963, played a major role. New meteor showers began to grow at a rapid pace, refining the mean orbits of known meteor showers at that time, both from photographic observations and from radar observations. At this time, the main authors of the study of meteor showers were: C. S. Nilson (1964), B. L. Kashcheyev a V. N. Lebedinets (1967), L’. Kresák a V. Porubčan (1970), B. A. Lindblad (1971), Z. Sekanina (1973, 1976), A. F. Cook (1973), G. Gartrell a W. G. Elford (1975), A. K. Terentjeva (1989), V. Porubčan a M. Gavajdová (1994), etc.
Research of meteor showers today
Due to the massive growth of video technology over the past 30 years, there has been a significant increase in the number of multi-station meteor orbits (or meteoroid orbits). Hand in hand with this trend, of course, is rapidly increasing the number of newly discovered meteor showers. At present, the following databases of meteoroid orbits are available: CAMS (Cameras for Allsky Meteor Surveillance), IMO VMN (International Meteor Organization Video Meteor Network), SonotaCo, EDMOND (European viDeo MeteOr Network Database), CMN (Croatia Meteor Network), IAU MDC (Photographic Meteor Orbits Database), etc. The highest number of newly discovered meteor showers comes from CAMS database (P. Jenniskens; 1994, 2006, 2012-2014, 2016) and CMN (Šegon; 2012-2015). The increase in the number of newly discovered meteor showers is shown in the picture below, and before 2005, it was mostly the refinement of the mean orbits of already known meteor showers, not the discovery of new showers with the exception of radio observations (e.g. Z. Sekanina, A. F. Cook, etc.) – here, on the contrary, they were mostly new meteor showers active in the day, i.e. out of the reach of visual or photographic observers.
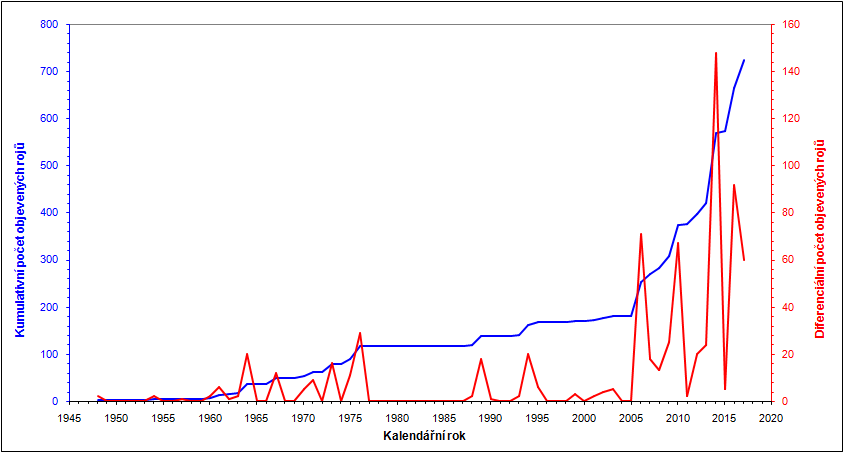
Fig. 1: Differential and cumulative numbers of discoveries of new meteor showers from 1948 to 2017. Author: Jakub Koukal
At first glance, it may seem that a huge increase in the number of newly discovered meteor showers was caused only by the development of observation techniques. Looking closer, however, it is obvious that the current methods for detecting of the new meteor showers are failing, and a high number of controversial cases can be found in the flood of new discoveries.
Methodology for discovery of new meteor showers
New meteor showers can be searched using so-called independent clustering. Here is possible dual approach, either all meteors will be included in the calculation, irrespective of their meteor showers – so all orbits are seen as sporadic or only sporadic meteors will be included in the calculation – so all meteors belonging to already known showers are excluded in advance. The first approach is more appropriate, it is possible to calculate the new mean orbits of already known showers without affecting the original mean orbit, which has been calculated in the past. The so-called orbit similarity criteria are used to assign individual meteor orbits. Basic criteria include only orbital elements, such as the Southworth-Hawkins (Southworth and Hawkins, 1963) criterion (DSH), the Drummond (Drummond, 1981) criterion (DD) or the Jopek (Jopek, 1993) criterion (DJ) other criteria also bring in the observed quantities (geocentric velocity, radiant position, etc.) – e.g. the Valsecchi (Valsecchi et al., 1999) criterion (DN). A sample of equations for calculating the Southworth-Hawkins criterion (DSH) is shown in Figure 2.
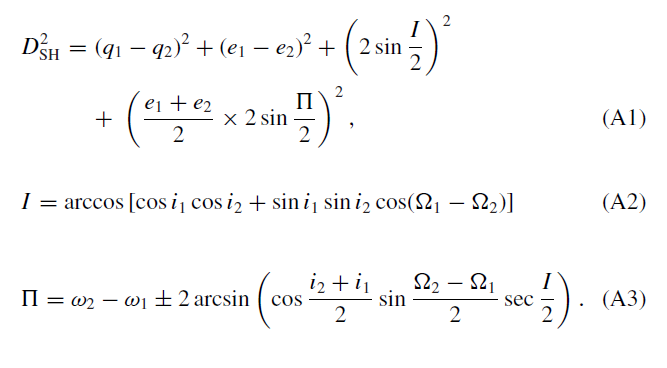
Fig. 2: Equations for calculating the Southworth-Hawkins criterion of orbits similarity (DSH). Author: Southworth, Hawkins
All criteria have their limitations and are, for example, sensitive to low-slope orbits and also have different maximum values for differentiating the sporadic background from meteor showers. Increasing the limit value of the criterion results in the assignment of sporadic meteor orbits to the meteor shower. If the meteor shower is strong and clearly defined, the differential count of the number of meteors decreases (or stagnates) with the increasing value of the similarity criterion used. However, if the meteor is not clearly defined (for example, if it is a random cluster of sporadic meteors without a common origin) there is a steady increase in the number of orbits associated with the meteor shower. On this principle is based method “break-point” (Neslušan et al., 2013) which assesses the cumulative increase in the number of orbits in increasing the value of the criterion and also the differential increase in the number of orbits in the intermediate values of the criterion. With a sufficient number of shower orbits, the “core” of the meteor shower can be well defined by differential growth and the sporadic meteors can be distinguished by cumulative growth from shower meteors. The principle of the method for cumulative numbers of associated orbits is shown in Figure 3.
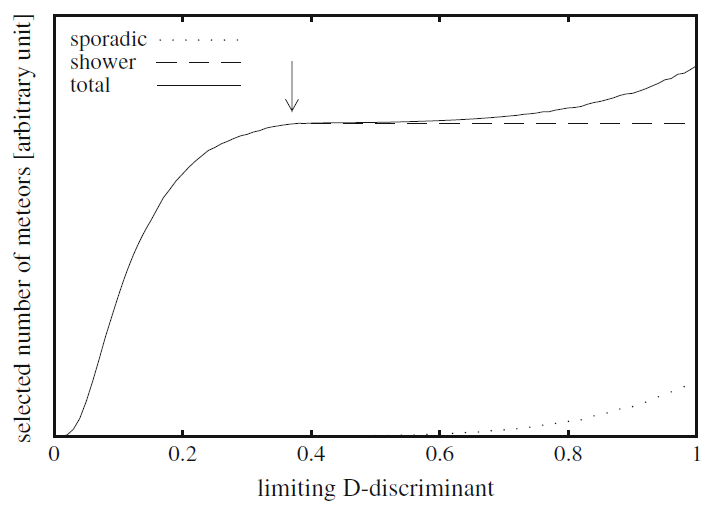
Fig. 3: The “break-point” method principle with the marked break point. With a further increase in the value of the similarity criterion, the sporadic background is assigned to the meteor shower. Author: Neslušan et al.
The practical use of the “break-point” method can be demonstrated in the strongest regular meteor shower, on Perseids. Using Drummond’s criterion of orbits similarity (DD), was detected through the EDMOND (Kornoš et al., 2014a,b) database that the width of the “core” of the Perseid meteor shower corresponds to the limit value DD < 0.03 and the total width of the meteor shower activity corresponds to the limit value DD < 0.17. Figure 4 also shows clearly how the shower “core” is defined (the differential orbit count) and how this increase in differential orbit counts stagnates with increasing of DD criterion.
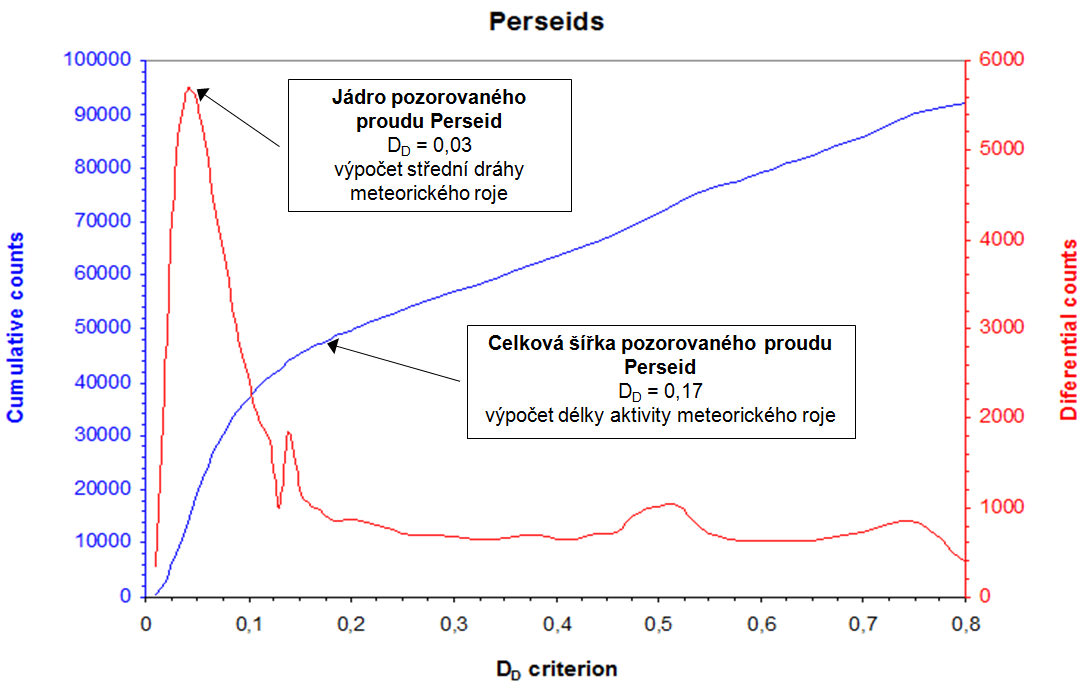
Fig. 4: Practical use of the “break-point” method for the Perseid meteor shower (007 PER). Values of the DD criteron for the shower “core” and for its width (activity) are highlighted. Author: Jakub Koukal
Meteor showers IAU MDC database
The IAU MDC meteor showers database (Jopek et al., 2014) contains all the information that are currently available for all classified meteor showers. In addition to data on the period of activity also includes the orbital elements of mean orbits of meteor showers (if known), the radiant position, its daily motion, geocentric velocity, and a source with a number of orbits that served to calculate the orbital elements of meteor showers mean orbits. The IAU MDC showers database currently contains 726 meteor showers, of which only 112 are established and 26 are in the unconfirmed (pro tempore) category, 545 meteor showers from this list have been discovered/added over the last 12 years.
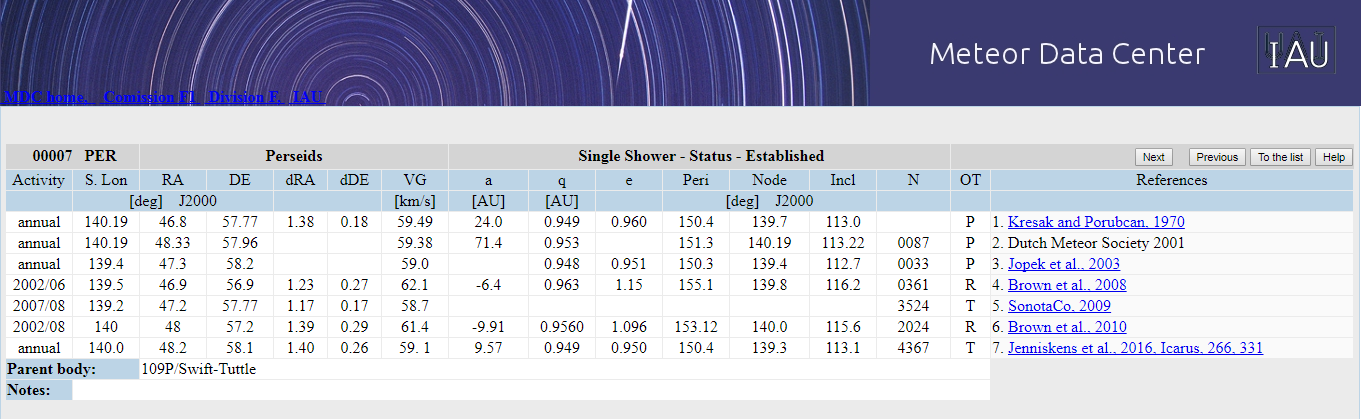
Fig. 5: Overview of mean orbits from individual sources for the Perseid meteor shower (007 PER), including listing of individual sources in the IAU MDC database. Author: IAU MDC
For known and strong meteoric showers (e.g. Perseids, Geminids, Leonids, etc.), the amount of sources for calculating the mean orbit is considerable. A relatively large part of the meteor showers has, of course, a mean orbit defined by only one source while the number of orbits for the calculation is quite often relatively small, sometimes the calculation is made from less than 10 orbits. Anyway, the amount of meteor showers is currently higher than the total number of individual orbits of photographic meteors 30 years ago.
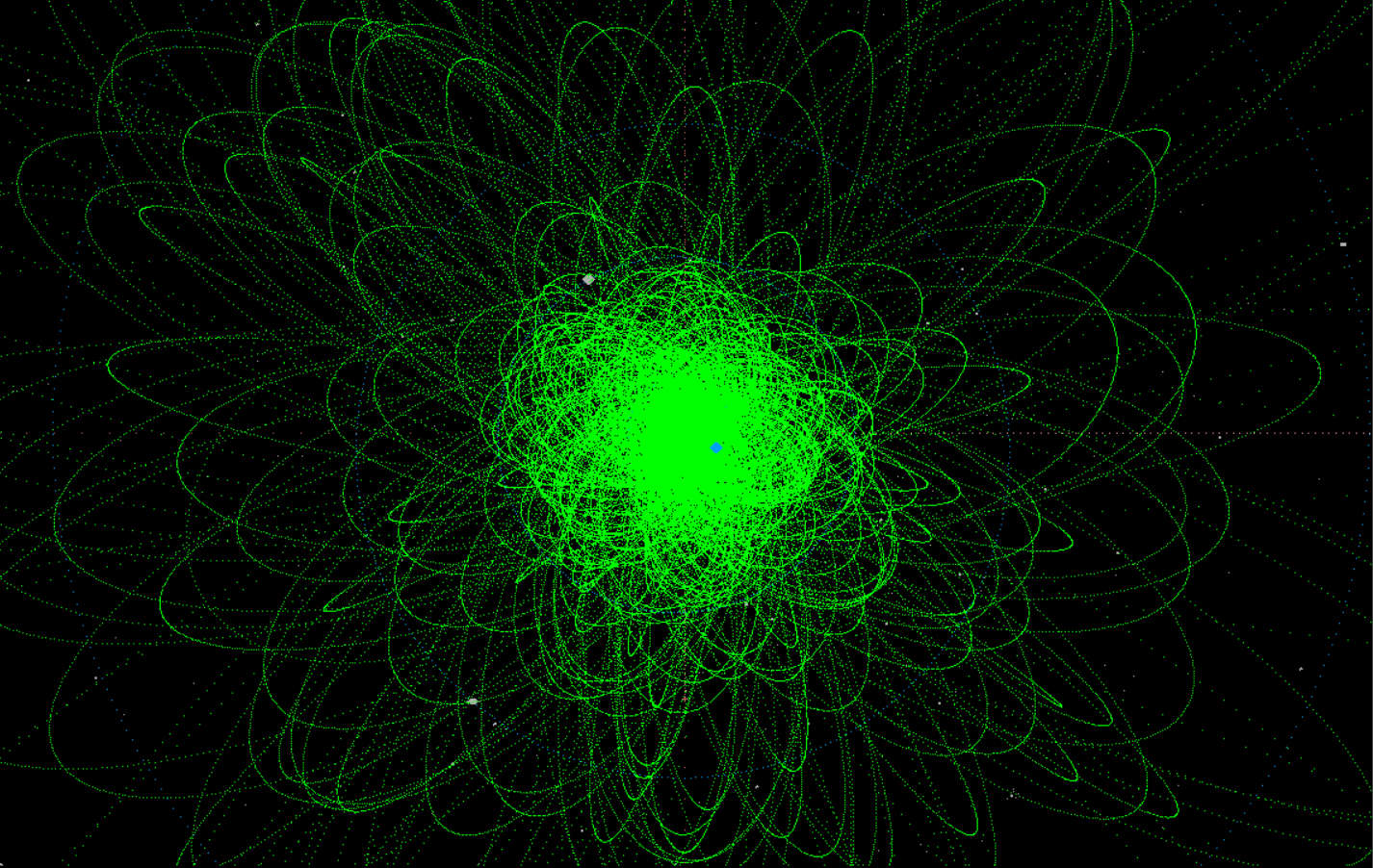
Fig. 6: Mean orbits of all 726 meteor showers in the IAU MDC database in side view of the center of the Solar System (the position of the planet Earth is marked in blue). Author: Jakub Koukal
Division of known meteor showers
A typical and very complex case is the Taurid complex, or the 2P/Encke comet complex. To massive and complex system with very long activity include besides the southern (002 STA) and northern (017 NTA) Taurids many smaller showers (e.g. the northern and southern delta Piscids, northern and southern October delta Arietids, etc.), but also two powerful daily showers – beta Taurids and zeta Perseids which occur at the second intersection (node) of the orbit of the complex and the Earth’s orbit. Taurid complex division into sub meteor showers attempts to capture the variety and quantity of parent bodies which, like the 2P/Encke comet, originated in the past by the collapse of the massive original cometary body. In addition, the overall situation will make the gravitational perturbation of planets difficult, especially Jupiter, and also to the fact that this is the largest mass flow in the inner part of the solar system. The search for asteroids that can be associated with the complex or the search for the filaments of the complex has been devoted to and will probably be devoted to a number of scientific works in the future.For example (Porubčan et al., 2006) mentions 7 filaments in the complex and 9 associated NEO asteroids (2001 HB, 2003 SF, 2001 QJ96, 1999 RK45, 2003 QC10, 2003 WP21, 2004 TG10, 2003 UL3, 2003 WP21 a 2002 XM35) of which 4 (2003 QC10, 2004 TG10, 2003 UL3 and 2002 XM35) were evaluated in this paper as the most probable parent bodies for individual filaments found. Also (Spurný et al., 2017) talks about the newly discovered branch of the complex in connection with the increased activity of bright bolides of the complex in 2015. In this work, 3 asteroids are associated with the Taurid complex (2015 TX24, 2005 UR and 2005 TF50).
Table 1 gives an overview of the mean orbits of the new meteor showers from the IAU MDC catalog in relation to the major showers of the Taurids complex (002 STA and 017 NTA) including orbital elements, and the Drummond criterion for the similarity of the orbits in relation to both major showers. Some new meteor showers are associated with previously mentioned asteroids (e.g. 630 TAR – 2005 TF50, 632 NET – 2004 TG10) however, for example meteor showers Taurids (628 STS) is associated directly with the 2P/Encke comet and due to the low value of the DD criterion in relation to the southern Taurids mean orbit (DD = 0.035), it will not be possible to reliably assign the individual meteors to mean orbit of s Taurids shower.
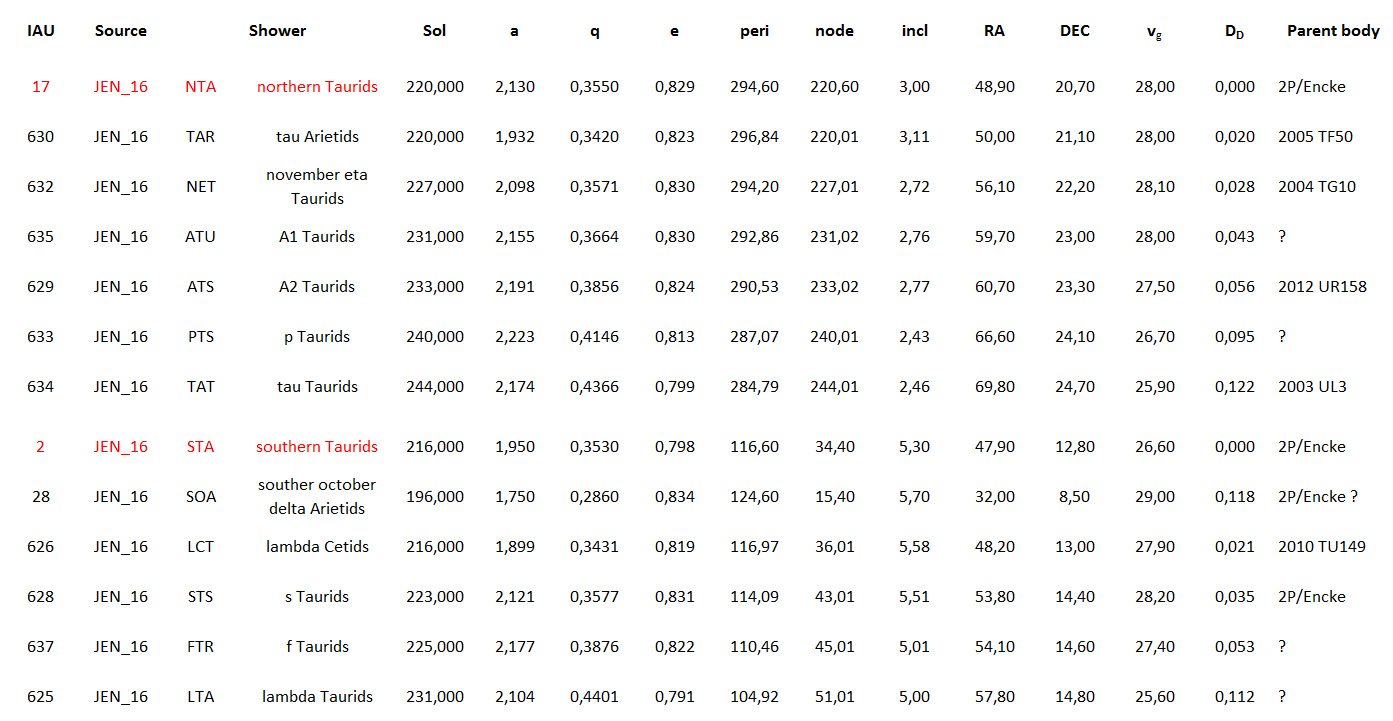
Tab. 1: Overview of the mean orbits of new meteor showers from the IAU MDC catalog in relation to the major showers of the Taurids complex (002 STA and 017 NTA).The used orbital elements of the mean orbits are taken from (Jenniskens et al., 2016). The column “DD” denotes the value of the Drummond criterion for the similarity of the orbits in relation to the main showers of the complex (STA, NTA), at the same time, the parent body is specified if known (Jenniskens et al., 2016). Author: Jakub Koukal
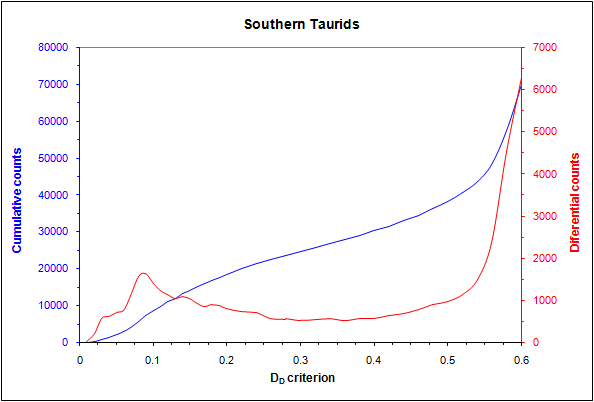
Fig.7: Graph of differential and cumulative counts of meteor shower 002 STA members for increasing value of DD criterion („break-point“ method). The graph shows the complexity of the Taurids complex, the similarity of the meteor orbits and the position of other complex filaments, including the meteoric shower 017 NTA. Author: Jakub Koukal
The core of the meteor shower usually falls within the range of 0.03 < DD < 0.05, the width ofactivity of meteor shower is usually between 0.15 < DD < 0.20, for scattered meteoric showers it may be even higher. And the Taurids complex is exactly this case, due to its development and gravitational perturbations from Jupiter. The same problem is visible for several other meteor showers, such as 630 TAR (0.020), 632 NET (0.028), 635 ATU (0.043), and 629 ATS (0.056), the mean orbits of which are very close to the mean orbit of the major shower 017 NTA. The same problem also occurs for the new showers 626 LCT (0.021) and 637 FTR (0.053) relative to the mean orbit of the second major shower 002 STA. The mean orbits of all these meteor showers, including southern and northern Taurids, are shown in Figure 8. Therefore, it would be more appropriate in these cases to talk about the branches or filaments of the two major showers of the complex and not of the new meteor showers.
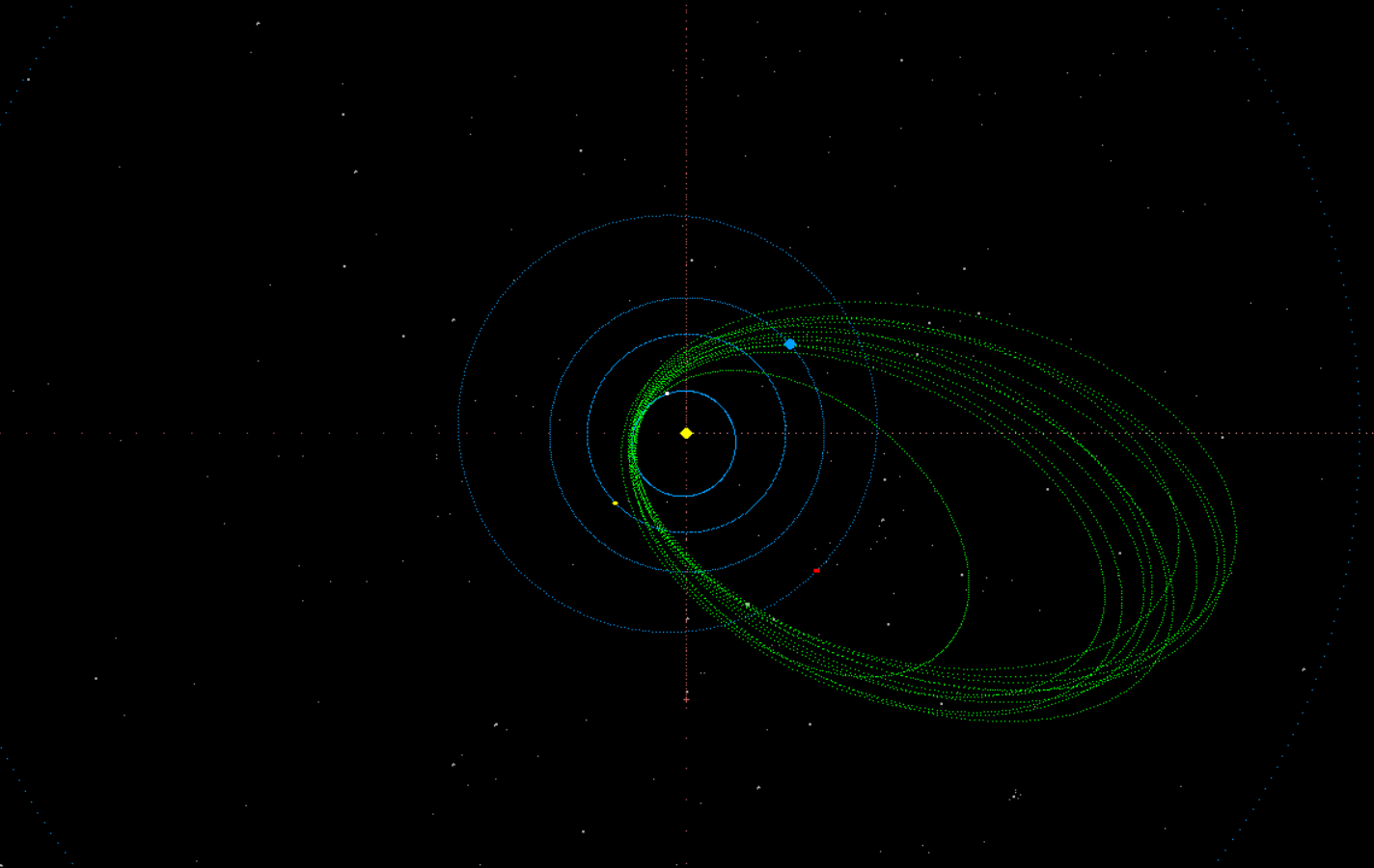
Fig. 8: The mean orbits of all 13 meteor showers listed in Table 1 whose orbital elements are very similar (DD<0.15) to the orbital elements of the main showers of the complex (002 STA and 017 NTA). Author: Jakub Koukal
New meteor showers on a strong sporadic background
A typical representative of this group is the kappa Cepheids meteor shower (751 KCE, Šegon et al., 2015). For the mean orbit of the shower mentioned in the IAU MDC database, 17 meteors were used by source work. However, given the relatively high geocentric velocity (vg = 33.7 km/s), the total area of individual meteor radiants is relatively large, the radiant dimension in the declination exceeds 10°. Using the Drummond criterion DD was found in the database EDMOND 29 orbits for DD < 0.05, 441 orbits for DD < 0.10 and even 1354 orbits for DD < 0.15.
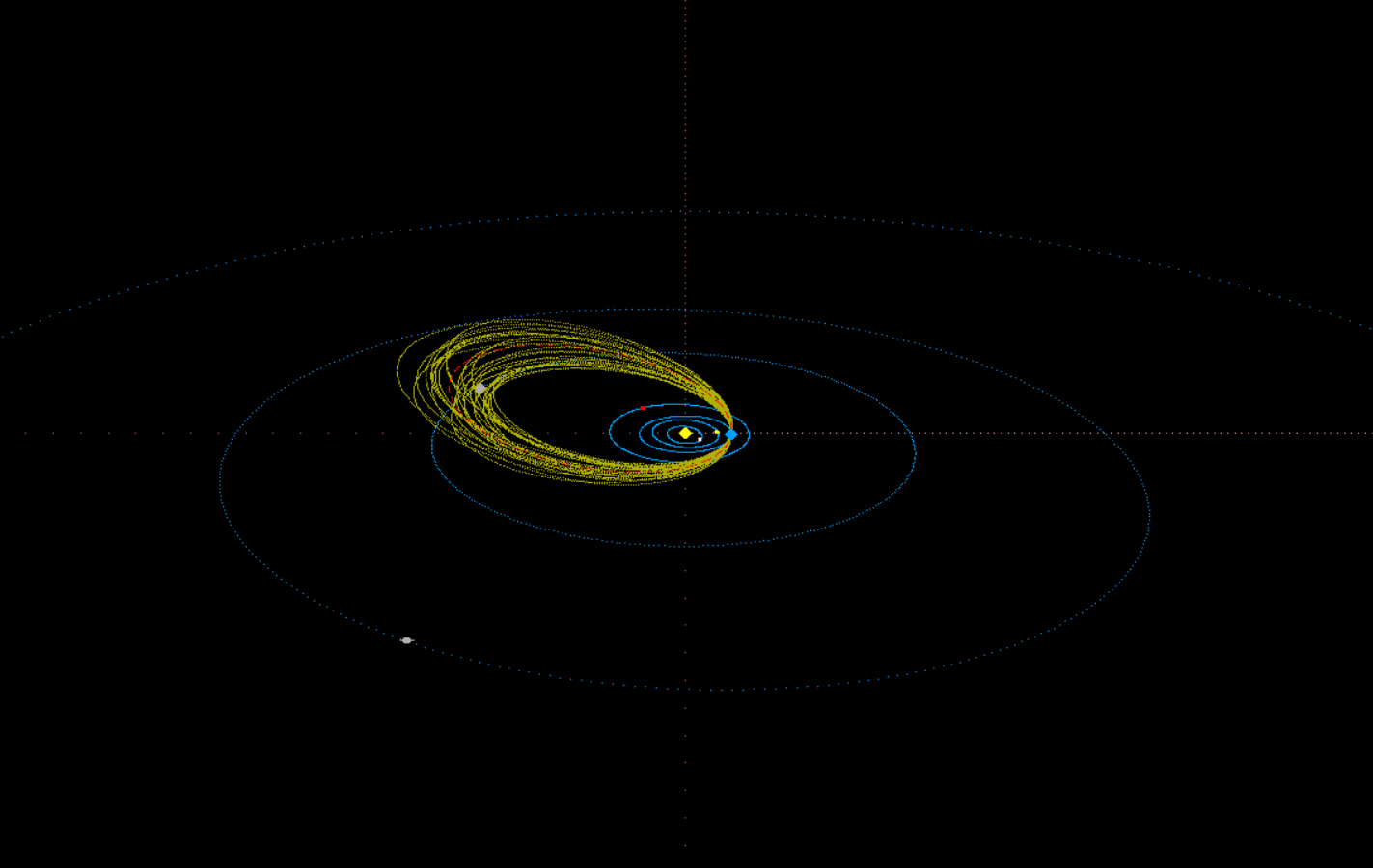
Fig. 9: The orbits of 29 meteors assigned to meteor shower 751 KCE with the DD criterion value < 0.05. Author: Jakub Koukal
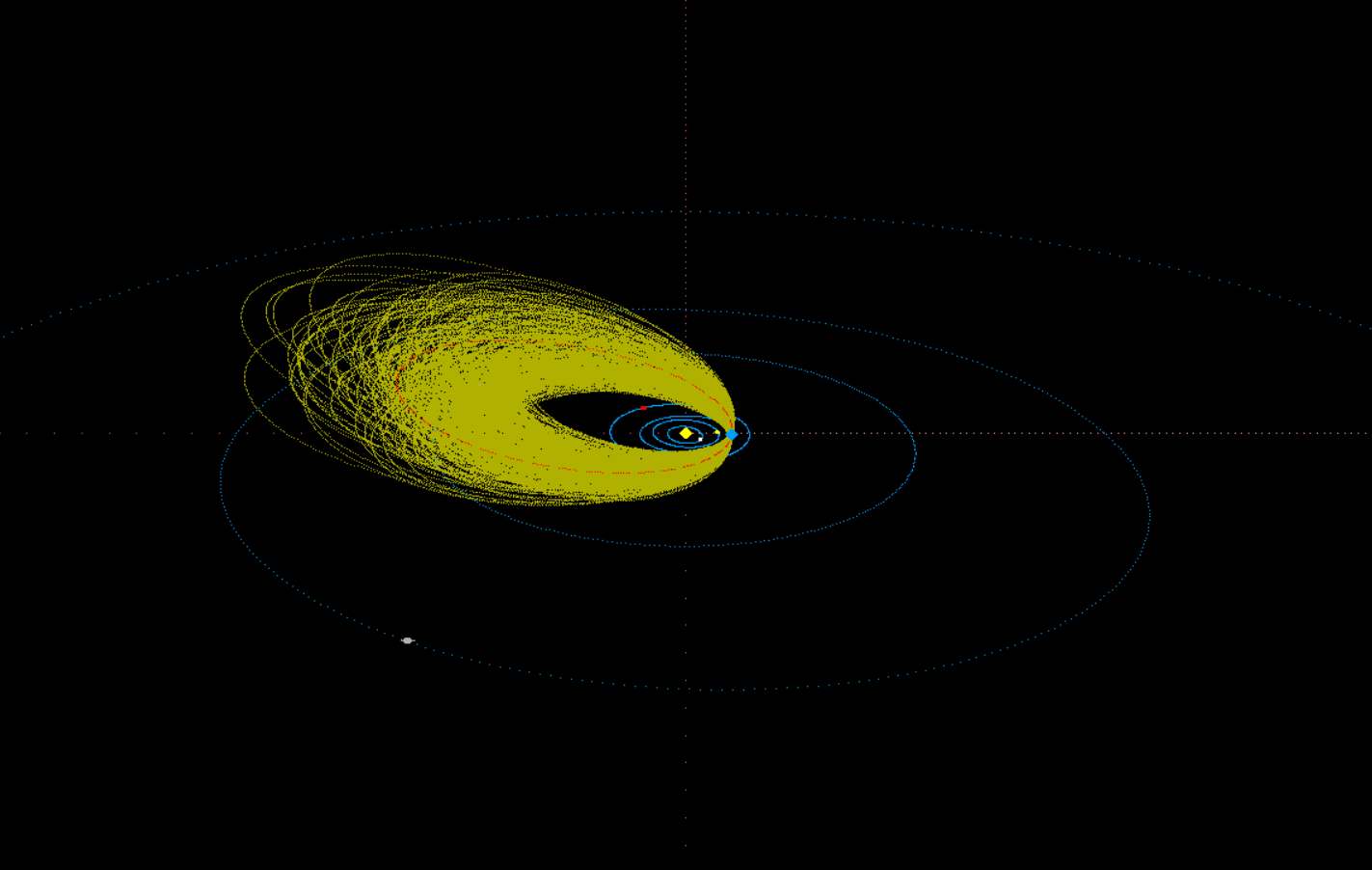
Fig. 10: The orbits of 441 meteors assigned to meteor shower 751 KCE with the DD criterion value < 0.10. Author: Jakub Koukal
The graph of the differential and cumulative counts of numbers of 751 KCE shower members is shown in Figure 11 and shows a steep increase in cumulative meteor counts assigned to the mean orbit of the shower with increasing value of the DD criterion.Because of this, it seems that in the case of the meteor shower kappa Cepheids, these meteors are only randomly selected orbits from the sporadic background, or the densification of the sporadic background at the site of the supposed shower. The existence of this meteor shower is therefore highly controversial.
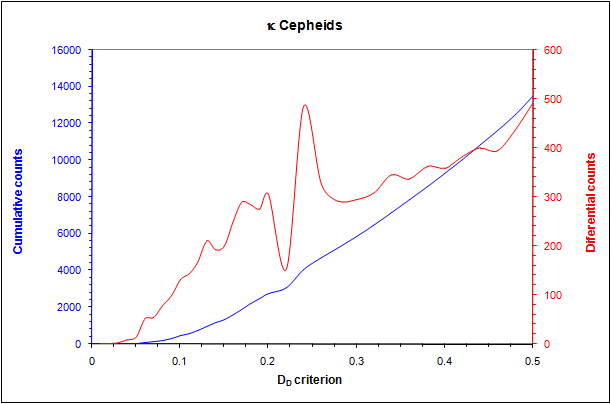
Fig.11: Graph of differential and cumulative counts of numbers of751 KCE shower members for increasing value of DD criterion („break-point“ method). The graph shows a steep increase in cumulative counts of meteor shower numbers, as well as individual peaks in differential counts of meteor numbers corresponding to nearby meteor showers, or other areas with a higher sporadic background density. Author: Jakub Koukal
Double meteor showers on a strong sporadic background
In this case, it is a combination of the problem of the Taurids complex and the problem of the kappa Cepheids. The IAU MDC database already has a meteor shower (in this case phi Draconids – 45 PDF) and the newly discovered shower (psi Draconids – 754 POD) has a mean orbit very similar to the original shower. In addition, both are located in the area of the toroidal complex, which contains a large number of sporadic meteors, and the existence of any meteor shower here is very difficult to prove. Basically, the number of meteor showers found in this area depends only on the criteria selected for the selection and results in a certain number of mean orbits of “showers” of very similar orbits, which are only rotated in the length of the perihelion. The mean orbit of the shower 754 POD has a Drummond criterion DD = 0.048 in relation to the mean orbit of shower 45 PDF. This again means (as with Taurids) the problem of assigning individual meteors to both showers within the considered width of showers activity, the mean orbits of both meteor showers are shown in Figure 12.
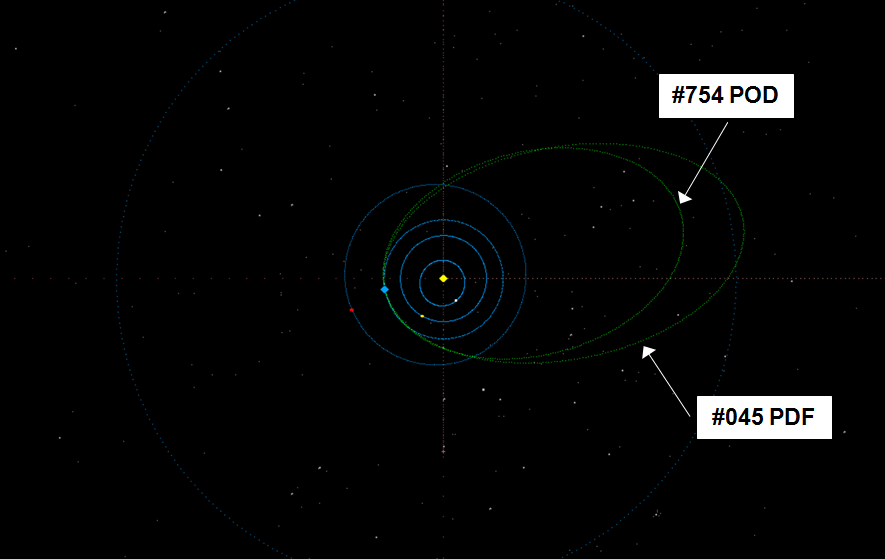
Fig. 12: Mean orbits of meteor showersphi Draconids (45 PDF) and psi Draconid (754 POD). Author: Jakub Koukal
For the mean orbit of the shower mentioned in the IAU MDC database, 31 meteors were used by the source work (Šegon et al., 2015). Using the Drummond DD criterion, EDMOND found 77 orbits for DD < 0.05, 493 for DD < 0.10, and even 1038 for DD < 0.15. The graph of the differential and cumulative counts of the 754 POD shower meteors (as well as the shower 45 PDF) is shown in Figure 13 and shows a steep increase in the cumulative counts of meteors assigned to the mean orbit of the shower with the increasing value of the DD criterion. Because of this, it seems that in the case of meteor shower psi Draconids are only randomly chosen orbits from a sporadic background, eventually the concentration of the sporadic background at the site of the supposed swarm. The existence of this meteor swarm is therefore highly controversial. The same of course applies to shower 45 PDF (Jenniskens et al., 2006), which has a very similar mean orbit and it is rotated only in the length of the perihelion.
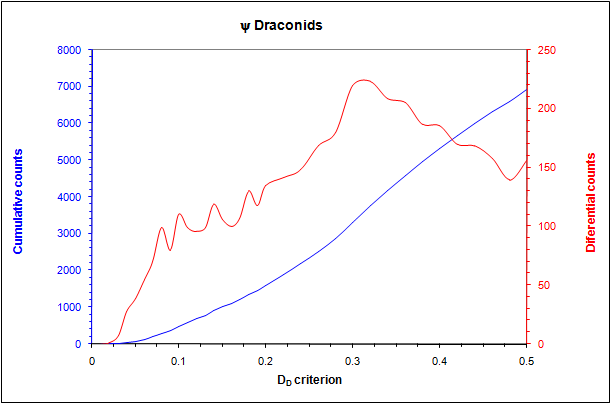
Fig.13: Graph of differential and cumulative counts of numbers of754POD shower members for increasing value of DD criterion („break-point“ method).The graph shows a steep increase in cumulative counts of meteor shower numbers, as well as individual peaks in differential counts of meteor numbers corresponding to nearby meteor showers, or other areas with a higher sporadic background density. Author: Jakub Koukal
Conclusions
Although this article could be construed as being critical, it was not thus intended. The author, on the contrary, very much values the work of all the above, and only points out the problems that arise within the massive development of this part of astronomy science. However, due to the above problems, the revision of the IAU MDC catalog appears to be necessary. The minimum (and first step) should be the “clustering” of all meteors in the databases without affecting the mean orbits of the already known meteor showers. Also, where possible, the dynamic development of individual orbits in the past (reverse orbit integration) should be considered. With the increasing number of orbits recorded, of course, the need for analyzes is increasing, and the current methodology, even with the use of new procedures (Welch, 2001), fails to solve all the problems arising from the huge input data.
References
Cook A.F. (1973). A working list of meteor streams. NASA Special Publication, 319, 183.
Drummond J. D. (1981). A test of comet and meteor shower associations. Icarus, 45, 545-553.
Jenniskens P. (1994). Meteor stream activity I. The annual streams. Astronomy and Astrophysics, 287, 990-1013.
Jenniskens P. (2006). Meteor showers and their parent comets. Cambridge University Press
Jenniskens P., Nénon Q., Albers J., Gural P.S., Haberman B., Holman D., Morales R., Grigsby B.J., Samuels D.,Johannink C. (2016). The established meteor showers as observed by CAMS. Icarus, 266, 331-354.
Jopek T. J. (1993). Remarks on the meteor orbital similarity D-criterion. Icarus, 106, 603-607.
Jopek T. J., Kanuchova Z.(2014).Current status of the IAU MDC Meteor Shower Database.Proceedings of the Meteoroids 2013 Conference, Aug. 26-30, 2013, A.M. University, Poznań, Poland, eds. Jopek T.J., Rietmeijer F.J.M., Watanabe J., Williams I.P., A.M. University Press, 2014, 353-364.
Gartrell G., Elford W.G. (1975). Southern hemisphere meteor stream determinations. Australian Journal of Physics, 28, 591-620.
Holman D., Jenniskens P. (2012). Confirmation of the Northern Delta Aquariids (NDA, IAU\# 26) and the Northern June Aquilids (NZC, IAU\# 164). J. Int. Meteor. Org, 40, 166-170.
Holman D., Jenniskens P. (2013). Discovery of the Upsilon Andromedids (UAN, IAU\# 507). WGN, Journal of the International Meteor Organization, 41, 43-47.
Kashcheyev B.L., Lebedinets V.N. (1967). Radar studies of meteors. Smithsonian contributions to astrophysics, 11, 183.
Kornoš L., Koukal J., Piffl R., Tóth J. (2014a). EDMOND Meteor Database. In Gyssens M. and Roggemans P., editors, Proceedings of the International Meteor Conference, Poznań, Poland, Aug. 22-25, 2013. IMO, 23–25.
Kornoš L., Matlovič P., Rudawska R., Tóth J., Hajduková M. Jr., Koukal J., Piffl R. (2014b). Confirmation and characterization of IAU temporary meteor showers in EDMOND database. Proceedings of the Meteoroids 2013 Conference, Aug. 26-30, 2013, A.M. University, Poznań, Poland, eds. Jopek T.J., Rietmeijer F.J.M., Watanabe J., Williams I.P., 225-233.
Kresák L’, Porubcan V. (1970). The dispersion of meteors in meteor streams. I. The size of the radiant areas. Bulletin of the Astronomical Institutes of Czechoslovakia, 21, 153.
Lindblad B.A. (1971). A computerized stream search among 2401 photographic meteor orbits.. Smithsonian Contributions to Astrophysics, 12, 14-24.
Porubčan V., Gavajdová M. (1994). A search for fireball streams among photographic meteors. Planetary and Space Science, 42, 151-155.
Southworth R. R., Hawkins G. S. (1963). Statistics of meteor streams.Smithson. Contrib. Astrophys. 7, 1963, 261-286.
Lindblad B. A., Neslušan L., Porubčan V., Svoreň J. (2003). IAU Meteor Database of photographic orbits version 2003. Earth, Moon, Planets, 93, 249–260.
Neslušan L., Svoreň J., Porubčan V. (2013). The Method of Selection of Major-Shower Meteors Revisited. Earth, Moon, and Planets, 110, 41-66.
Nilsson C.S. (1964). A southern hemisphere radio survey of meteor streams. Australian Journal of Physics, 17, 205-256.
Porubčan V., Kornoš L., Williams I. P. (2006). The Taurid complex meteor showers and asteroids. Contributions of the Astronomical Observatory Skalnate Pleso, 36, 103-117.
Rudawska R., Jenniskens P. (2014). New meteor showers identifed in the CAMS and SonotaCo meteoroid orbit surveys. Meteoroids 2013, 217-224.
Sekanina Z. (1973). Statistical model of meteor streams III. Stream search among 19303 radio meteors. Icarus, 18, 253-284.
Sekanina Z. (1976). Statistical model of meteor streams. IV. A study of radio streams from the synoptic year. Icarus, 27, 265-321.
Spurný P., Borovička J., Mucke H., Svoreň J. (2017). Discovery of a new branch of the Taurid meteoroid stream as a real source of potentially hazardous bodies. Astronomy & Astrophysics, 605, A68.
Šegon D., Andreič Z., Korlevič K., Gural P., Novoselnik F., Vida D., Skokič I. (2012). New shower in Cassiopeia. WGN, Journal of the IMO, 40, 195-200.
Šegon D., Andreič Z., Korlevič K., Novoselnik F., Vida D., Skokič I. (2013). 8 new showers from Croatian Meteor Network data. WGN, Journal of the International Meteor Organization, 41, 70-74.
Šegon D., Gural P., Andreič Z., Skokič I., Korlevič K., Vida D., Novoselnik F. (2014). New showers from parent body search across several video meteor databases. WGN, Journal of the IMO, 42, 57-64.
Šegon D., Gural P., Andreič Z., Vida D., Skokič I., Novoselnik F. (2015). Four possible new high-declination showers. WGN, Journal of the IMO, 43, 147-150.
Terentjeva A.K.(1989). Fireball streams. Asteroids, Comets, Meteors III, 579.
Valsecchi G. B., Jopek T. J., Froeschlé C. I. (1999). Meteoroid stream identification: a new approach—I. Theory. Monthly Notices of the Royal Astronomical Society, 304, 743-750.
Welch P. G. (2001). A new search method for streams in meteor data bases and its application. Monthly Notices of the Royal Astronomical Society, 328, 101-111.