By Tammo Jan Dijkema, Denis Vida, Cees Bassa, Nick Moskovitz and Peter Eschman
Abstract: Using the cameras of the Global Meteor Network in Arizona, Colorado and New Mexico we reconstruct the reentry trajectory of the Dragon capsule trunk (Crew-5 flight) which occurred on 2023-04-27 around 08:52 UTC. We compare the preliminary trajectory to known orbital information of the object and deduce the moment of rapid reentry.
Introduction
On 11 March 2023, four astronauts returned from the International Space Station in the Crew-5 Dragon spacecraft. Shortly before the capsule reentry, it separated from its 37 m3 trunk. The trunk entered an orbit around Earth, and reentered on 27 April 2023, around 08:52 UTC. The reentry of the trunk was well observed by meteor cameras of the Global Meteor Network (GMN) in the American Southwest. We report on these observations and compare the camera-derived trajectory with the last known orbit of the trunk.
Observations
The reentry was observed by 21 GMN cameras. All of them had clear skies, allowing accurate astrometric calibration on many stars. The reentry was detected on almost all cameras by the fireball detector and raw video of the reentry was saved. For some parts of the observations, we had to fall back to “FF” files in the ‘four-frame format’ (Vida et al., 2021) which contain data aggregated over 10 seconds. An overview of the observations is shown in Figure 2.
Figure 1 shows the cameras that observed the reentry over time. The majority of cameras that observed the event were operated by either the Lowell Observatory or the New Mexico Meteor Array (NMMA).
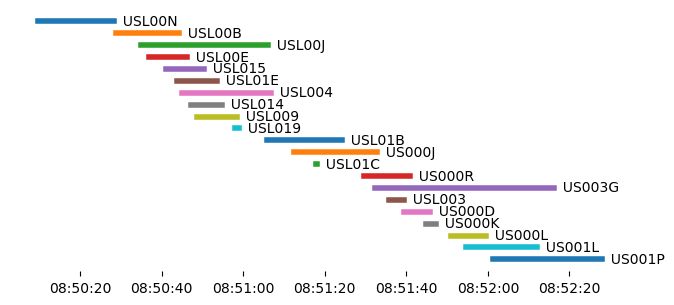
Figure 1 – Cameras contributing over time (UTC)
Data reduction
All observations were manually calibrated and the location of the trunk on every video frame was manually picked using the SkyFit2 software (Vida et al., 2021). These picks are available in the Global Fireball Exchange (GFE) format and are part of the accompanying data release (Dijkema et al., 2023).
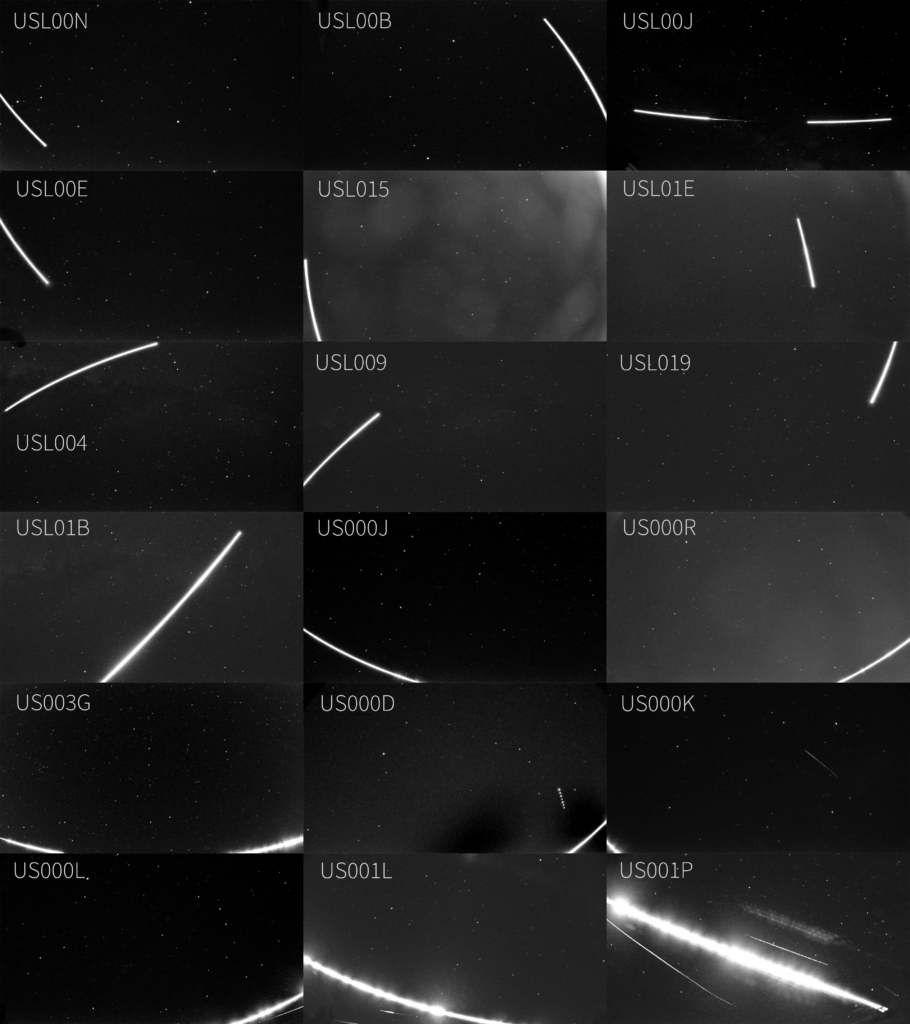
Figure 2 – Co-added images of the camera observations. Note that only portions of the trajectory are shown from some stations for which FF files were available.
The trajectory was computed piecemeal using the WesternMeteorPyLib (WMPL) trajectory solver (Vida et al., 2019). The solver was developed and tuned for meteors on heliocentric orbits which have a much shorter duration and higher speeds, unlike satellites on geocentric orbits. When used without alterations, the solver will produce an inaccurate trajectory with high deviations from the measurements.
We have worked around the limitations of WMPL by cutting the data into time chunks, where each time chunk is treated as an individual event. This technique introduces some discontinuities in the trajectory, which have been marked in the figures. Essentially, the trajectory solver does not compensate for the lift experienced by the spacecraft which curves the trajectory but assumes that individual pieces of the trajectory are straight lines. The compensation for the trajectory curvature due to gravity was also disabled as the lift significantly reduced the amount of trajectory deviation. The same technique was used previously in the reduction of a StarLink reentry above Spain in February 2022 (Dijkema et al., 2022).
Comparison with the last-known orbit
Most commonly, the orbits of satellites are monitored by radar systems such as the US Space Surveillance Network (SSN). The orbital parameters are shared on space-track.org in the form of two-line elements (TLEs). Typically, these orbital elements are updated daily.
The last known TLE of the Dragon trunk, with a NORAD ID 55840, has epoch 2023-04-27T07:40. The epoch is not the time of the radar measurement, but it should be close to it. This means that the orbital parameters were measured using radar observations only hours before the reentry. For an object this close to reentry, the accuracy of the TLE orbit is low.
The ground track of the propagated TLE is shown as a red line in Figure 3. It matches our trajectory well. A deviation is visible in the first part of the trajectory which is likely due to the inaccuracy of the meteor observations: only one camera observed the beginning (see Figure 1).
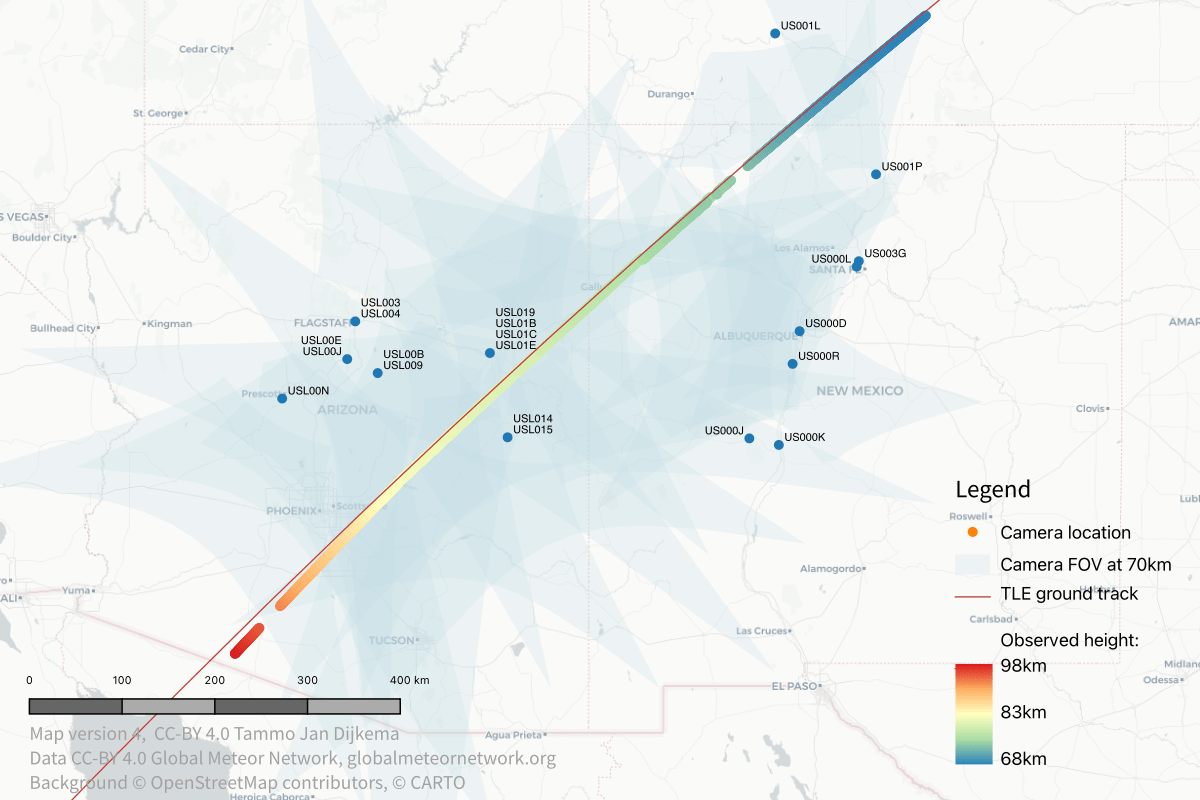
Figure 3 – Ground track of the reentry. The red line shows the ground track of the propagated TLE orbit.
Our observations show that the object is ahead of the predicted ground track. At the beginning of our observations, it is ahead of the TLE by about 7 seconds and at the end, it is ahead by about 4 seconds (due to obvious deceleration). It should be noted that there may be a time delay of our observations of about 0.5 seconds.
The main difference between the last known orbit and the observed trajectory is the height. Predicting a suitable ‘drag’ term in TLEs is notably hard because this term depends on many factors like the orientation of the spacecraft and the solar activity (determining the extent of the atmosphere). The TLE orbit predicts a height of 134 km at the time of the reentry. The observations show (Figure 4, top panel) an actual height of around 98 km down to around 68 km (the beginning and end of the trajectory are less accurate).
The TLE orbit predicts an almost constant velocity of 7.8 km/s around the time of the observed reentry. Our observations do not lead to a good velocity fit until 08:50:40. From then on, we observed a velocity of 7.6 km/s decreasing to 6.2 km/s (Figure 4, bottom panel). The trajectory was not observed in full; the trunk exited the field of view of the last camera before the end.
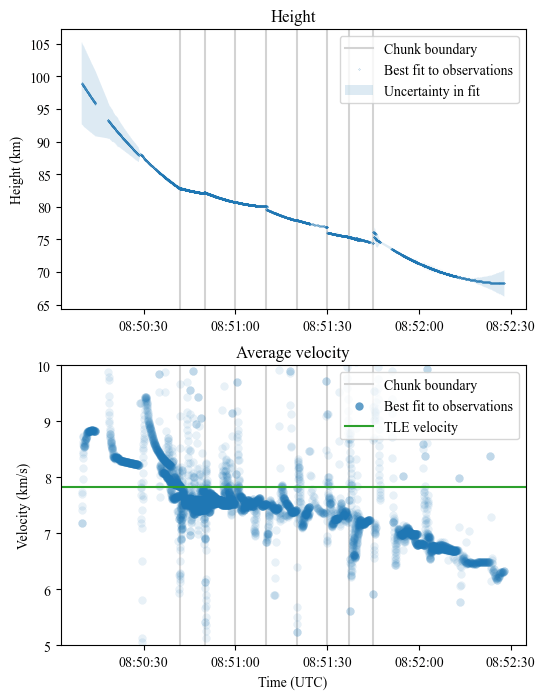
Figure 4 – Top: height vs. time of the observed trajectory. The TLE height is not in the plot, it is at 134 km. Bottom: velocity vs. time our observed trajectory. Fitting errors before around 08:50:40 are present as only one camera observed the beginning.
Future work
To improve the trajectory, a trajectory solver could be used which includes the last known orbital information and computes the drag and lift dynamics. From past meteoroid work, a solver that could be considered is that of Sansom et al., 2019. From the spacecraft side, a solver could be used in NASA’s General Mission Analysis Tool (Hughes et al., 2017).
Conclusion
In this preliminary analysis, we have shown that Global Meteor Network observations of the Crew-5 trunk reentry match the orbit of the Crew-5 trunk well and that meteor camera observations can provide valuable information about the timing, location, height, and dynamics of satellite reentries.
Acknowledgment
We thank all camera operators of the involved cameras for contributing their data: Thomas Blog, Solvay Blomquist, Bob Broffel, Jim Fordice, Matt Francism, Megan Gialluca, John Glitsos, Bob Greschke, Sam Hemmelgarn, Gene Mroz, David Robinson, Robert Schottland, Jay Shaffer, Eric Toops and Steve Welch.
References
Dijkema, T. J., Arce, E., Carballada, J., Martín, J. L., & Jordán Sánchez, F. (2022). Global Meteor Network observations of Starlink re-entry 2022-02-10. Zenodo. https://doi.org/10.5281/zenodo.6123485
Dijkema, T. J., Vida, D., Moskovitz, N., & Eschman, P. (2023). Global Meteor Network observations of Crew-5 Dragon trunk re-entry 2023-04-27. Zenodo. https://doi.org/10.5281/zenodo.7904716
Hughes, S. P., Conway, D. J., Parker, J. (2017). Using the general mission analysis tool (GMAT), NASA Technical Report Server, GSFC-E-DAA-TN39043.
Sansom, E. K., Jansen-Sturgeon, T., Rutten, M. G., De-villepoix, H. A., Bland, P. A., Howie, R. M., … & Hartig, B. A. (2019). 3D meteoroid trajectories. Icarus, 321, 388-406.
Vida D., Šegon D., Gural P. S., Brown P. G., McInty-re M. J., Dijkema T. J., Pavletić, L., Kukić, Ma-zur, M.J., Eschman P., Roggemans P., Merlak A., Zubrović, D. (2021). “The Global Meteor Network – Methodology and first results”. Monthly Notices of the Royal Astronomical Society, 506, 5046–5074.
Vida, D., Gural, P. S., Brown, P. G., Campbell-Brown, M., & Wiegert, P. (2019). Estimating tra-jectories of meteors: an observational Monte Carlo approach–I. Theory. Monthly Notices of the Royal Astronomical Society, 491(2), 2688-2705.