Abstract: A possible new meteor shower had been recently reported based on single station camera images. The apparent radiant position was located near the star rho Ser with approximately coordinates at R.A. = 238º; Dec. = +18º on 13–14 August 2020, about: λʘ = 141.2º. A search among 1.3 million video meteor orbits resulted in a dataset with 89 meteors from this radiant area, with a fair number of orbits fulfilling the discrimination criteria. However, no trace of any concentration could be detected. This case study proves that caution is required with impressions based on single station meteor observations. Meteoroid stream searches using D-criteria require caution too, especially for low inclination short orbits near the ecliptic, D-criteria may produce false positives.
1 Introduction
When watching the starry sky during a Perseid display some other, less active meteor showers manage to attract the attention of the visual observer. Every now and then a few sporadic meteors may confuse the observer when it looks like if these meteors come from a common area at the sky, suggesting a possible unknown meteor shower radiant. The random appearance of meteor trails at the sky has been often very misleading for single station observers. The only certainty a single station observer has, is that the radiant of the meteor is somewhere on its backwards produced trail. In case of a major meteor shower the radiant area will become visible as the area where many backwards produced trails cross each other at the sky. This makes it possible to assume the shower identification with a fair chance to have it right.
Unfortunately, the obvious effect of backwards produced intersections, which makes sense only in case of significant statistical numbers of events, was assumed to be also suitable to locate minor shower radiants. This way, the often randomly intersecting backwards produced meteor trails were assumed to define minor shower radiants which was the beginning of a long controversial polemic. It started in the 19th century with visual observers plotting meteor trails with chalk on a black stellar globe, later gnomonic star maps were used. Visually observing fast short-lived events like meteors is a real challenge for human eyes and brains. The plotting errors were believed to be rather small while in practice the errors on the direction of the trail as well as the beginning and ending points made the meteor plotting work questionable. Some visual observers from the past believed their eyes had a recording capacity and precision comparable to modern video meteor cameras while their brain flowless solved the complex geometrics which are today computed by powerful computers and carefully designed software applications. This resulted in long lists with discovered minor meteor streams, most of which were just fake, illusions created by randomly intersecting meteor trails combined with plotting errors.
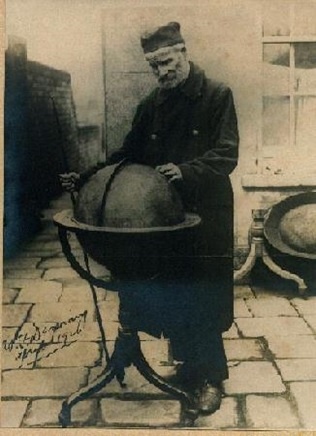
Figure 1 – William Frederick Denning in the early 20th century with his black stellar sphere on which meteors were plotted with chalk.
When dedicated photographic and radar meteor observing campaigns revealed a quite different picture of the radiant distribution in the 1950s, the past visual observing efforts to define minor shower radiants lost credibility and with it, unfortunately all visual meteor work became unpopular. It took until the 1970s before a new generation of amateur astronomers resumed visual meteor observing, initially making the same assumptions and mistakes like in the late 19th, first half of the 20th century, but most amateurs soon understood the limitations imposed by human eyes and brains. Visual work focused on recording activity levels of major showers, based on statistical relevant numbers of events. However, some amateurs continued to believe in visual meteor plotting to determine minor shower radiants. Computer programs were made to visualize the hot spots of meteor intersections but at some point, it was understood that plotting errors diffused the picture. The problem with these plotting errors was solved when video meteor recording became affordable to amateurs. Instead of using multiple station video meteor work, the unfortunate mistake has been made to use single station data for shower identification, applying the same late 19th century methodology for visual observed plotted meteor trails on modern video recordings. The random pure chance intersections again produced plenty of fake minor shower candidates, making up long lists of spurious radiants. When video meteor observing got applied based on multiple station scale, permitting triangulations to determine the trajectory, radiant and orbit, the radiant distribution and existence of minor showers could finally be verified.
2 Video observational data
Never before the sky has been better guarded for meteor activity and fireballs than during past few years with several dedicated video camera networks operated worldwide. Daylight and bad weather can still interfere and prevent detection of unexpected short lived meteor showers, but anything active during several nights or recurrent during different years should have been observed by video cameras. Therefore, it is worthwhile to check if any activity has been detected of the low velocity Serpentids (Velkov, 2021).
In our orbit database we have 471582 orbits obtained by CAMS (Oct.2010–Dec.2016, Jenniskens et al., 2011), 663031 orbits obtained with UFOCapture (2006–2020, EDMOND, SonotaCo network, Kornoš et al., 2014; SonotaCo, 2009) and 191393 orbits obtained by GMN (Oct. 2018– Feb. 2021, Vida et al., 2021). Altogether, we have 1326006 orbits available.
In order to limit our search sample, we select all orbits collected around the time of the Serpentids appearance, a time bin of 10° in solar longitude centered at λʘ = 141°. 118843 orbits are available within this interval. The apparent radiant was given in equatorial coordinates at α = 238° and δ = +18° with a low velocity vg < 15 km/s. Typical for meteor showers with such low velocity is that the zenith attraction has a great effect especially when the radiant is low, resulting in a huge radiant area. We select all radiants in within 15° around right ascension and declination, roughly a radiant with a diameter of 30° to account for the large spread in the apparent radiant positions. Only 89 orbits had their radiant in this area in the selected interval 136° < λʘ < 146°, all of these had vg < 15 km/s, since there aren’t any fast meteors coming from this part of the sky where meteoroids have to hit the rear side of the Earth compensating the speed of Earth in its orbit around the Sun. With 89 meteors with the right speed coming from the suspected radiant area any single station meteor observer would be happy to confirm the existence of the rho Serpentids, statistically this looks like a relevant number of events. What about the orbits, are these orbits similar?
3 Comparing the orbits
In order to consider all the orbits with the same criteria an iterative procedure has been applied, using the mean orbit of the 89 selected orbits as the reference orbit to identify all similar orbits that may define the meteor shower. This method has been described before (Roggemans et al., 2019).
To calculate a reference orbit for a collection of similar orbits we do not use the median or average values of the orbital elements, but we compute the mean orbit according to the method described by Jopek et al. (2006). To compare orbits on similarity researchers established different discrimination criteria, often abbreviated as D-criteria. The D-criteria that we use are these of Southworth and Hawkins (1963), Drummond (1981) and Jopek (1993) combined. The oldest and most popular D-criterion, the one established by Southworth and Hawkins or DSH proved often too tolerant and unsuitable for short period low inclination orbits near the ecliptic. It is not unusual that orbits which are very similar according to DSH, fail for another D-criteria such as that of Drummond or DD.
Taking the mean orbit of the selected 89 orbits as initial reference orbit, an iterative procedure recomputes the mean orbit for the orbits with D-criteria within a chosen threshold relative to the reference orbit. To limit the risk for pure chance similarity we took: DSH < 0.1 & DD < 0.04 & DH < 0.1 as upper limits. The iterative procedure converges after a number of iterations with an orbit which is best representative as mean orbit for 27 orbits which respect the above-mentioned D-criteria:
- αo = 237.9°
- δo = +20.4°
- αg = 225.7°
- δg = +12.9°
- vg = 8.4 km/s
- a = 2.23 AU
- q = 1.0105 AU
- e = 0.553
- i = 6.1°
- ω = 174.9°
- Ω = 141.1°
At this point we could shout “hurray” we confirmed the suspected new shower of the rho Serpentids. As many as 53 orbits respect the less strict D-criteria with DSH < 0.25 & DD < 0.105 & DH < 0.25 which is often used in literature as acceptable to assume similarity between the orbits. On the other hand, 36 meteors which had their radiant in the suspected radiant area with the right speed fail in the D-criteria. Any single station observer, either a visual observer or any single station recording system would have counted all these meteors as rho Serpentids as coming from the right direction with the expected low velocity.
However, caution is required with this kind of low inclination short period orbits. Although we have a nice number of orbits fulfilling the similarity criteria, this only indicates that the orbits have similarity, it does not prove there is any physical relationship between these orbits. With only four almost identical orbits (DSH < 0.05 & DD < 0.02 & DH < 0.05), it looks like there is no real concentration of these orbits.
4 Misleading D-criteria?
The D-criteria are helpful to check for similarity between orbits which is a useful criterium for most meteoroid streams with higher inclination, Jupiter family comet orbits or Halley comet orbits. The method can be very misleading for short period low inclination orbits as the Solar system is full of sporadics with such orbits near the ecliptic. To define a meteor shower it is not enough to find just similar orbits according to D-criteria, there must be a concentration visible in radiant positions are among the orbital elements.
For any single station observer all our selected orbits would have produced a rho Serpentid candidate, coming from the right radiant area with the expected speed. Looking at the plot of all 89 apparent radiant positions (Figure 2) we see a rather wide scatter. The black dots are radiants for orbits that fail in the D-criteria, the blue dots have low similarity and the red dots high similarity. Unfortunately, there is nothing that looks like a concentration of radiants as should be the case if there is a real meteor shower. The black, blue and red dots appear randomly distributed.
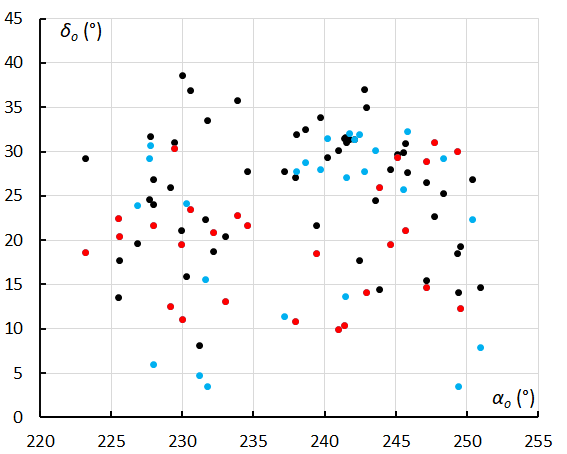
Figure 2 – The apparent radiant positions in equatorial coordinates, color coded with black for no orbit similarity, blue for weak orbit similarity and red for strong orbit similarity.
The apparent equatorial radiant positions suffer a large spread due to the Earth gravitation which has a large effect on slow velocity meteoroids especially when the zenith distance of the radiant is large. A more appropriate way to look at meteor shower radiant concentrations is to look at the plot of the computed Sun-centered geocentric ecliptic coordinates. By taking the difference between the ecliptic longitude λ and the solar longitude λʘ we can neutralize the radiant drift due to the Earth’s motion around the Sun. The result is shown in Figure 3. Still, there is no trace of anything like a concentration of radiants.
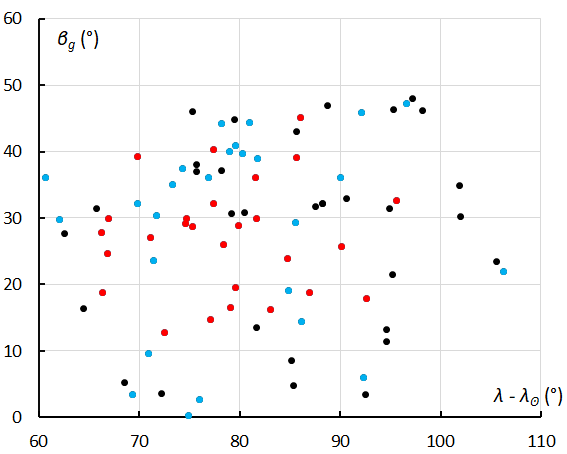
Figure 3 – The radiant positions in Sun-centered geocentric ecliptic coordinates, color coded with black for no orbit similarity, blue for weak orbit similarity and red for strong orbit similarity.
Another way to look for concentrations is to look at the orbital elements, for instance a plot of the inclination i against the length of perihelion Π. The result is shown in Figure 4 and also here we see a random distribution of no similarity orbits, low and high similarity orbits, no trace of any concentration that could indicate the presence of a real meteor shower.
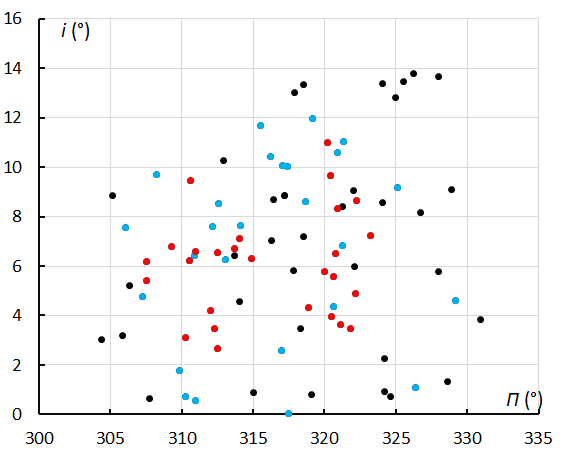
Figure 4 – The orbit inclination i against the length of perihelion Π, color coded with black for no orbit similarity, blue for weak orbit similarity and red for strong orbit similarity.
Looking for this type of orbits in the weeks ahead and after the suspected rho Serpentid activity, the same type of orbit appears, except for the ascending node which is time dependent, the same misleading positive matches for the D-criteria were found.
5 Conclusion
Whenever meteor observers report possible new shower activity, an in-depth study is required. Meteors and statistics make it pretty difficult to interpret visual impressions. When searching any kind of single station data sources, it will be easy to find meteor trails that confirm the impression. Thanks to the efforts of many amateurs and professional meteor workers worldwide a gold mine of meteor orbits became available. This way it became almost impossible for any new shower or unexpected meteor activity to escape attention.
The reported possible rho Serpentids situated in the western evening sky which produces only slow meteors heavily affected by the zenith attraction resulting in a huge radiant area are a nice example how misleading meteor appearances can be, especially for single station meteor work. But also using meteoroid orbits, caution is required as the available tools like D-criteria only indicate geometric similarity but do not prove any physical relationship between the orbits. If no concentration can be spotted in the radiant plots or orbital elements, then it is a case of pure statistical random coincidence, which is the case for the suspected rho Serpentids. We can safely conclude that there is no indication for the existence of the rho Serpentids.
References
Drummond J. D. (1981). “A test of comet and meteor shower associations”. Icarus, 45, 545–553.
Jenniskens P., Gural P. S., Grigsby B., Dynneson L., Koop M. and Holman D. (2011). “CAMS: Cameras for All-sky Meteor Surveillance to validate minor meteor showers”. Icarus, 216, 40–61.
Jopek T. J. (1993). “Remarks on the meteor orbital similarity D-criterion”. Icarus, 106, 603–607.
Jopek T. J., Rudawska R. and Pretka-Ziomek H. (2006). “Calculation of the mean orbit of a meteoroid stream”. Monthly Notices of the Royal Astronomical Society, 371, 1367–1372.
Kornoš L., Matlovič P., Rudawska R., Tóth J., Hajduková M. Jr., Koukal J. and Piffl R. (2014). “Confirmation and characterization of IAU temporary meteor showers in EDMOND database”. In Jopek T. J., Rietmeijer F. J. M., Watanabe J., Williams I. P., editors, Proceedings of the Meteoroids 2013 Conference, Poznań, Poland, Aug. 26-30, 2013. A.M. University, pages 225–233.
Roggemans P., Johannink C. and Cambell-Burns P. (2019). “October Ursae Majorids (OCU#333)”. eMetN, 4, 55–64.
SonotaCo (2009). “A meteor shower catalog based on video observations in 2007-2008”. WGN, Journal of the International Meteor Organization, 37, 55–62.
Southworth R. R. and Hawkins G. S. (1963). “Statistics of meteor streams”. Smithson. Contrib. Astrophys., 7, 261–286.
Velkov V. (2021). “A possible new meteor shower in August during the Perseids”. eMetN, 6, 472–479.
Vida D., Šegon D., Gural P.S., Brown P. G., McIntyre M. J. M., Dijkema T. J., Pavletić L., Kukić P., Mazur M. J., Eschman P., Roggemans P., Merlak A., Zubrović D. (2021). “The Global Meteor Network – Methodology and First Results”. Monthly Notices of the Royal Astronomical Society, 506, 5046–5074.