By Paul Roggemans, Damir Šegon and Denis Vida
Abstract: The Global Meteor Network recorded a distinct concentration of June Bootid orbits with most of the orbits collected at λʘ = 90.2°, or 2022, June 21 at 22h UT. Visual hourly rates were very low with a ZHR ~0.5. The radiant plot shows a compact core of very similar orbits embedded within a very large radiant area with very dispersed radiants. Comparing with other networks in previous years suggests that a concentration of very similar orbits like in 2022 may have occurred in 2016 and 2010.
Introduction
The parent comet of the June Bootids, sometimes referred to as the iota Draconids, was discovered by Jean-Louis Pons, a French astronomer (1761 – 1831), in 1809 and found again in 1858 by Friedrich August Theodor Winnecke, a German astronomer (1835 – 1897). No meteor shower was known or associated to this comet until 1916 when the famous British meteor observer William Frederick Denning (1848 – 1931) observed an outburst of meteors on June 28 from a radiant between Boötes and Draco. Denning (1916) concluded that Pons-Winnecke’s Comet of 1819-58 satisfied the conditions to be the parent body. The radiant was placed in the correct region and the date agreed. The shower was observed again in 1921 with moderately low hourly numbers except for one Japanese observer, Kaname Nakamura who observed this shower activity during several nights with best rates on July 3 with 153 meteors in 35 minutes. The shower activity consisted mainly in faint meteors with an average magnitude of +4.8. This observer was known to have an exceptional sensitive vision compared to other observers (Yamamoto, 1922).
King (1927) mentions a letter from Mr. V. Maltzev, the secretary of the meteor section of a Russian astronomy society about the observations of this shower in the Russian territories. More than 4000 meteor trails were recorded at Tashkent (todays capital of Uzbekistan) during end of June and beginning of July 1927. The maximum occurred on June 27 when hourly rates reached 500 from a radiant near ζ Ursa Major. 90% of these meteors were fainter than the 5th magnitude, confirming the observations made in 1921 at Kyoto in Japan.
Although attempts were made to observe this shower in later years, only very low rates were seen which could be explained as sporadic noise being lined up with the assumed radiant position. The comet orbit gradually evolved away from the Earth’s orbit and nobody expected any June Bootid activity anymore when unexpectedly a strong outburst of the June Bootids was seen in 1998 on June 27 (Velkov, 1999) with a peak ZHR of 250 ± 50 at λʘ = 95.69 ±0.01° (Jenniskens, 2006). Until then, no reliable orbit was known for the June Bootids, but two photographed meteors at Ondrejov Observatory in the Czech Republic allowed Pavel Spurny and Jiri Borovicka to compute a first accurate orbit on June 27.89102 UT. The obtained orbital parameters (all for equinox J2000.0) were:
- αg = 222.88 ± 0.16°
- δg= +47.60 ± 0.06°
- vg= 14.1 ± 0.4 km/s
- a = 3.3 ± 0.3 AU
- e= 0.69 ± 0.03
- q= 1.01577 ± 0.00005 AU
- ω= 183.65 ± 0.07°
- Ω= 96.04559 ± 0.00003°
- i= 18.4 ± 0.4°
This meteoroid was of the most fragile, typically cometary type IIIB (Spurny and Borovicka, 1998). The heliocentric orbit corresponds to a previous orbit of the comet 7P/Pons-Winnecke and is far off from the current parent body orbit (see Table 1).
Sergey Shanov and Sergey Dubrovsky predicted another return on 2004, June 23. Visual observers reported June Bootid activity from June 22, 20h UT until June 23, 23h UT with a peak activity and ZHR = 18 ± 2 at 10h UT (λʘ = 92.21°), in good agreement with the prediction (Jenniskens, 2006).
Mikiya Sato did dynamical simulations of the meteoroid stream until 2055 and showed that there will be no more future encounters with the stream (Jenniskens, 2006). It looked as there was no hope anymore to collect more orbit data for the June Bootids as the 1998 and 2004 returns came some years too early for the upcoming video camera networks. Or would the unpredictable nature of meteor showers bring some surprise in the future?
Table 1 – The dynamic evolution of 7P/Pons-Winnecke with the orbit at past returns and June Bootids orbits.
Comet’s return | a (AU) | e | q (AU) | Ω (°) | ω (°) | i (°) |
1892 | 3.24 | 0.726 | 0.887 | 105.6 | 172.2 | 14.5 |
1898 | 3.24 | 0.715 | 0.924 | 102.2 | 173.4 | 17.0 |
1909 | 3.26 | 0.702 | 0.973 | 100.6 | 172.3 | 18.3 |
1915 | 3.26 | 0.702 | 0.971 | 100.5 | 172.4 | 18.3 |
1921 | 3.31 | 0.685 | 1.041 | 99.2 | 170.3 | 18.9 |
1927 | 3.31 | 0.686 | 1.039 | 99.1 | 170.4 | 18.9 |
1933 | 3.33 | 0.670 | 1.102 | 97.5 | 169.3 | 20.1 |
1939 | 3.33 | 0.670 | 1.101 | 97.5 | 169.4 | 20.1 |
… | … | … | … | … | … | … |
2015 | 3.42 | 0.638 | 1.239 | 93.4 | 172.5 | 22.3 |
P. Spurny and J. Borovicka (1998) | 3.30 | 0.690 | 1.016 | 96.0 | 183.7 | 18.4 |
This paper | 3.11 | 0.674 | 1.014 | 89.5 | 185.6 | 18.2 |
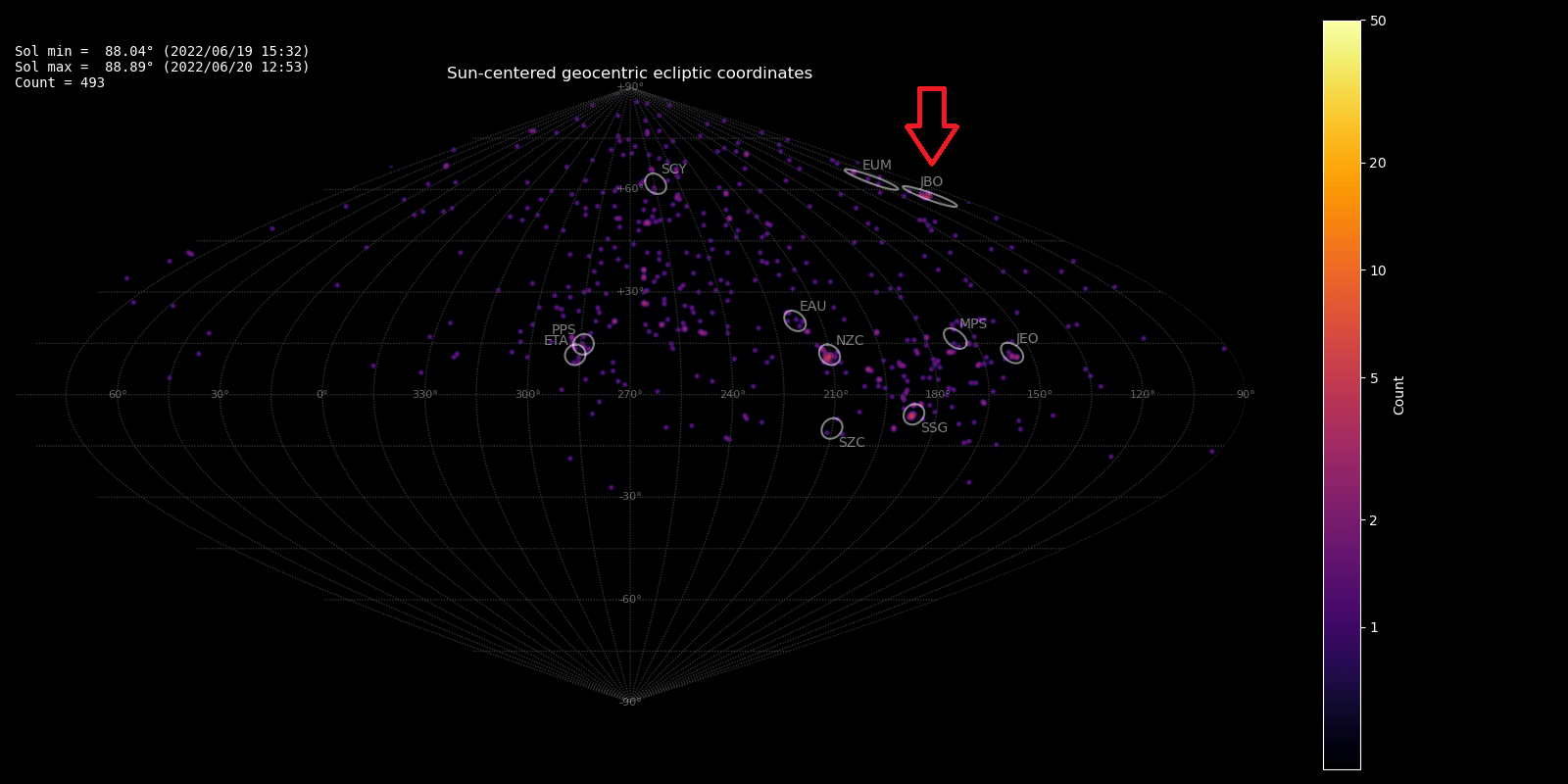
Figure 1 – The June Bootid radiant in Sun-centered geocentric ecliptic coordinates recorded by GMN during 88.04° < λʘ < 88.89° (June 19–20).
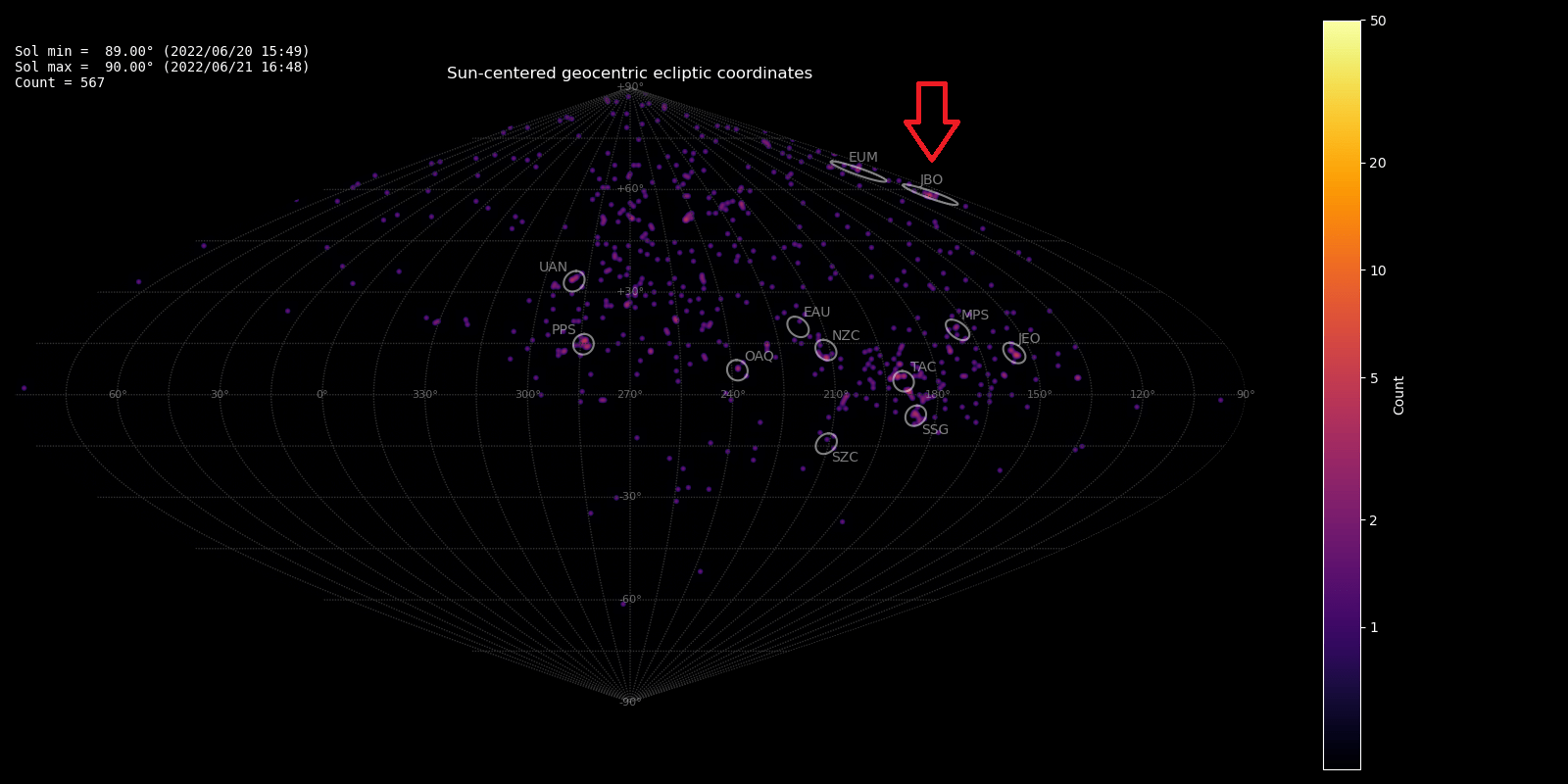
Figure 2 – The June Bootid radiant in Sun-centered geocentric ecliptic coordinates recorded by GMN during 89.00° < λʘ < 90.00° (June 20–21).
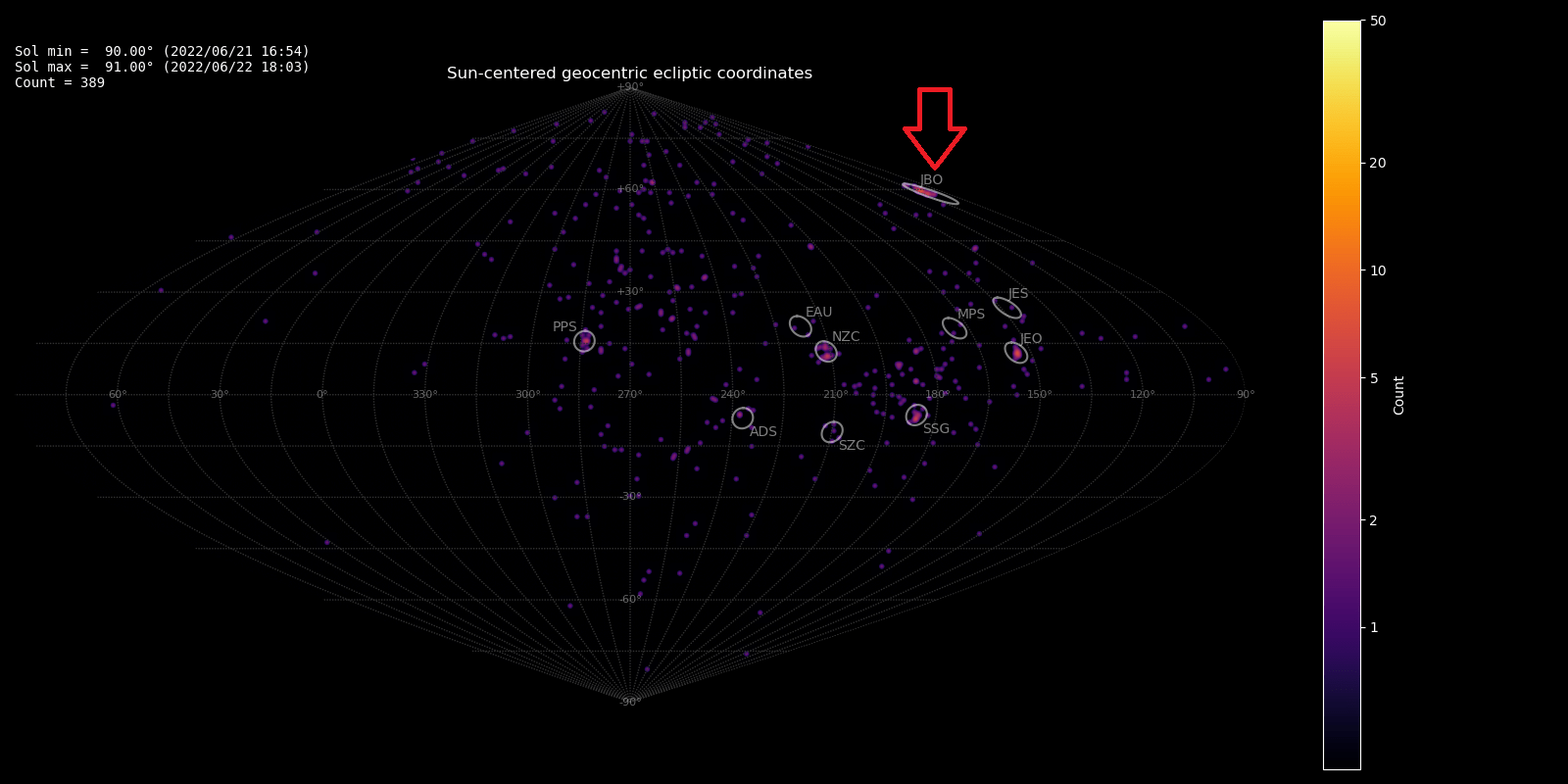
Figure 3 – The June Bootid radiant in Sun-centered geocentric ecliptic coordinates recorded by GMN during 90.00° < λʘ < 91.00° (June 21–22).
The GMN data
The JBO-radiant shows up in GMN radiant plots during three nights, 19–20, 20–21 and 21–22 June, nothing earlier or later (Figures 1, 2 and 3). The Global Meteor Network collected 2436 orbits during the five nights of 2022 June 18–23 (87.00° < λʘ < 92.00°) and identified 37 orbits as June Bootids (JBO#170) in 2022. A dense concentration of 31 orbits with DSH < 0.05 alerted the authors that some unusual activity occurred and required a dedicated analysis (Figure 4).
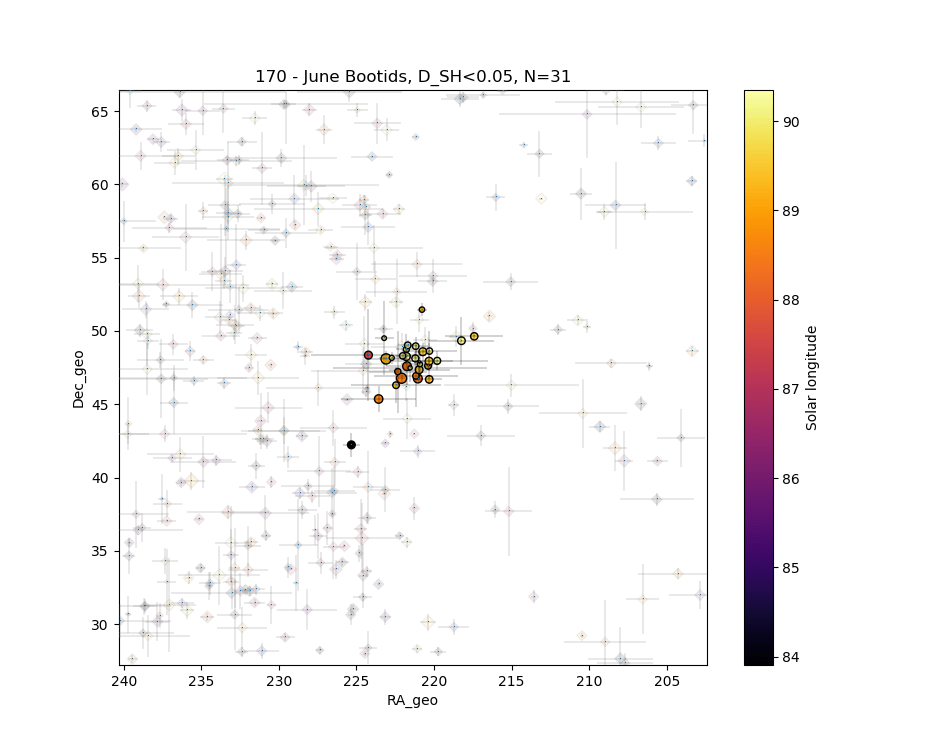
Figure 4 – Plot showing the concentration of radiants color coded in function of the solar longitude.
The initial identification by GMN has been made based on a list of known meteor showers (Jenniskens et al., 2018) for orbits recorded within 1° in solar longitude of the known activity period, with the radiant within 3° relative to the known radiant position and with a geocentric velocity vg within an interval of 10% relative to the reference geocentric velocity (Moorhead et al., 2020). Details about the methodology, theory and results of the Global Meteor Network can be found in Vida et al. (2019; 2020; 2021).
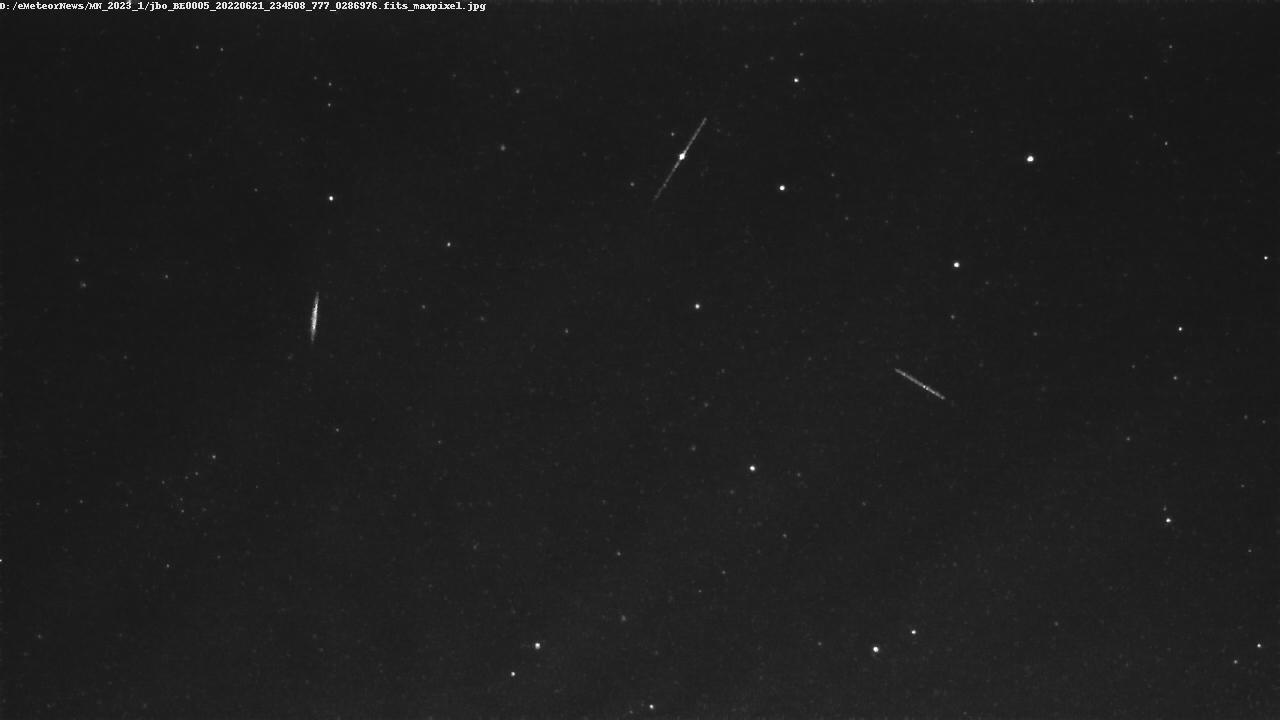
Figure 5 – June Bootid recorded on 2022 June 21, 23h45m12s on BE0005 at Grapfontaine, multi-station with BE0003 at Zillebeke, BE0008 at Genk and BE000C at Humain.
Most June Bootids were rather faint meteors, no really bright events. For instance, Figure 5 shows one of the brighter June Bootids recorded in 2022. The number of orbits identified as June Bootids by GMN in previous years is almost negligeable with only 3 in 2021, 5 in 2020 and none in 2019. It is unlikely that any unusual June Bootid activity was missed due to weather circumstances or lack of cameras as GMN covered the suspect period in previous years with a total of 254 orbits collected in 2019, 1079 in 2020 and 827 in 2021.
Using orbit similarity criteria, we find many more candidate June Bootid orbits within the suspect period: 8 in 2019, 57 in 2020, 20 in 2021 and 137 in 2022, most of these from a very dispersed radiant in equatorial coordinates as well as in Sun-centered ecliptic coordinates. Figure 6 shows the huge area at the sky where these widely dispersed radiants occur, most of them with very low or low similarity. Only in 2022 we have a distinct concentration of a statistically significant number of June Bootid orbits. The lower the velocity of a meteor shower, the larger the radiant tends to be. In such a case a dense concentration of radiants amid a widely spread radiant area indicates that Earth encountered some dust cloud of particles that didn’t get dispersed yet.
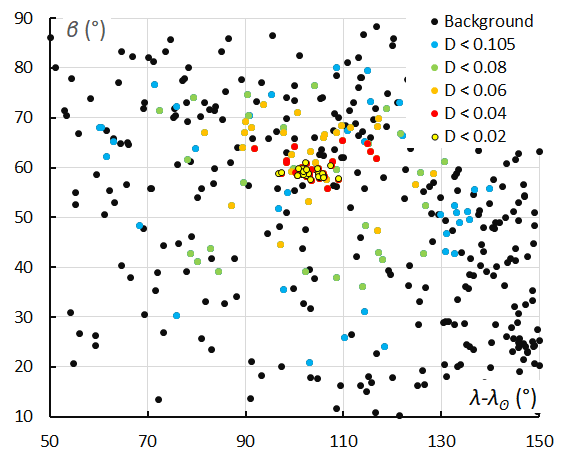
Figure 6 – The 2022 June Bootid radiants in Sun-centered geocentric ecliptic coordinates color coded for different classes of similarity.
Dispersed showers with large sized radiant areas are tricky to distinguish from the sporadic background and risk including sporadics. To eliminate these, we should look at the orbits after removing outliers that deviate too much from the mean orbit to avoid that the results get contaminated by ‘noise’. To search for JBO orbits, we selected our sample within the following intervals:
- 87° < λʘ < 92°
- 96° < λ –λʘ < 110°
- +54° < β< +64°
- 11 km/s < vg< 16 km/s
The radiant size considered corresponds to the concentration of very similar orbits in Figure 6. The GMN data in total has 43 orbits within these intervals for 2022. By limiting our search sample within this range in time and radiant size, we deliberately ignore June Bootids that appeared sooner or later as well as all cases with outlier radiant positions that may physically belong to this meteoroid stream, but which cannot be distinguished from the background activity on a statistical relevant basis. It may be worthwhile mentioning that these 43 orbits include three orbits identified as epsilon-Ursae Majorids (EUM#186), a meteoroid stream with orbital parameters that are very similar to the June Bootids and could be somehow related to it.
This sample has been searched with an iterative procedure to locate the best fitting mean orbit for a concentration of similar orbits. The method used for this has been described before (Roggemans et al., 2019a) and combines three classic discrimination criteria, considering different classes for the degree of similarity. The discrimination criteria used in this method are that of Southworth and Hawkins (1963), identified as DSH, Drummond (1981), identified as DD, and Jopek (1993), identified as DH. The method to compute the mean orbit during the iteration process has been described by Jopek et al. (2006).
We define five different classes with specific threshold levels of similarity:
- Low: DSH < 0.25 & DD < 0.105 & DH < 0.25;
- Medium low: DSH < 0.2 & DD < 0.08 & DH < 0.2;
- Medium high: DSH < 0.15 & DD < 0.06 & DH < 0.15;
- High: DSH < 0.1 & DD < 0.04 & DH < 0.1.
- Very high: DSH < 0.05 & DD < 0.02 & DH < 0.05.
These classes allow us to distinguish the degree of concentration and dispersion of the particles within the meteoroid stream. The iterative search identifies 42 orbits with low threshold similarity of which as many as 23 fit the very high threshold similarity. The mean orbits for each threshold class of similarity are listed in Table 2. These mean orbits were obtained for the sample limited to the core of the radiant shown in Figure 6. The values are almost identical regardless the class of similarity.
Table 2 – The mean orbits for the final selection of Global Meteor Network June Bootid orbits with five different threshold levels on the D-criteria.
Low | Medium low | Medium high | High | Very high |
|
λʘ | 89.69° | 89.71° | 89.69° | 90.22° | 89.64° |
αg | 221.6° | 221.7° | 221.6° | 221.2° | 221.5° |
δg | +48.1° | +48.1° | +48.1° | +48.0° | +47.9° |
vg | 13.9 | 13.9 | 13.9 | 13.9 | 13.9 |
λ – λʘ | 102.3° | 102.4° | 102.4° | 102.4° | 102.5° |
β | +59.1° | +59.2° | +59.0° | +58.9° | +58.8° |
Hb | 90.9 | 91.0 | 91.0 | 91.0 | 91.0 |
He | 80.7 | 80.5 | 80.5 | 80.8 | 80.5 |
a | 3.07 | 3.04 | 3.05 | 3.06 | 3.11 |
q | 1.0140 | 1.0140 | 1.0141 | 1.0142 | 1.0141 |
e | 0.6695 | 0.6659 | 0.6679 | 0.6688 | 0.6739 |
ω | 185.3° | 185.4° | 185.4° | 185.4° | 185.6° |
Ω | 89.57° | 89.58° | 89.58° | 89.70° | 89.48° |
i | 18.2° | 18.3° | 18.3° | 18.2° | 18.2° |
Π | 274.9° | 275.0° | 275.0° | 275.1° | 275.1° |
Tj | 2.78 | 2.80 | 2.79 | 2.78 | 2.76 |
N | 42 | 41 | 38 | 33 | 23 |
The number statistics are too small to compute a reliable meteor shower flux but based on the numbers and the calibrated time-area product, we can set an upper limit on the activity to a ZHR of ~0.5. We better look at the relative number of June Bootids as a percentage of the number of sporadic orbits in an interval of 0.2° in solar longitude (Figure 7). If we take all 137 possible JBO-orbits for 2022, including all these outliers obtained with the orbit similarity criteria, the result is heavily affected by these dispersed orbits (blue and green in Figures 6 and 7). There is a peak at λʘ = 90.2°, but this becomes more distinct when we limit our sample to the core of the radiant area where the orbit concentration appears (Figure 8). Most June Bootids were recorded at λʘ = 90.2°, or 2022, June 21 at 22h UT. In this interval alone, 9 of the 23 most similar JBO-orbits (yellow in Figure 8) were recorded. The number of sporadic orbits per time interval varied from 20 to 189 while some intervals had zero JBO-orbits recorded. Recalling the historic records of 1921 and 1927 when most meteors of the June Bootid outburst were +4 and fainter, it could be that our cameras captured only the brightest events as meteors of magnitude +4 and fainter are definitely below the detection limits of the cameras.
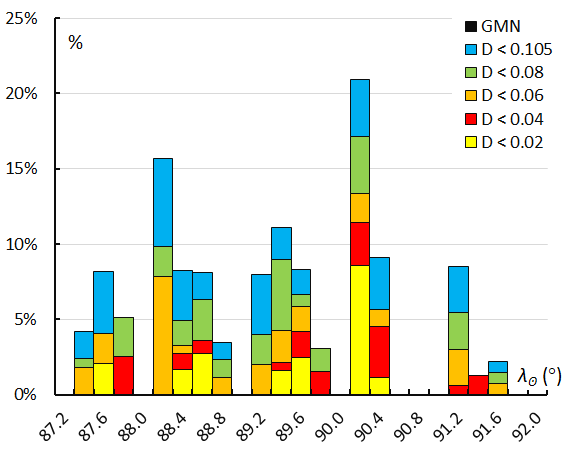
Figure 7 – The percentage of June Bootid orbits relative to the number of sporadic orbits in the a time interval of 0.2° in solar longitude for all possible JBO-orbits in 2022.
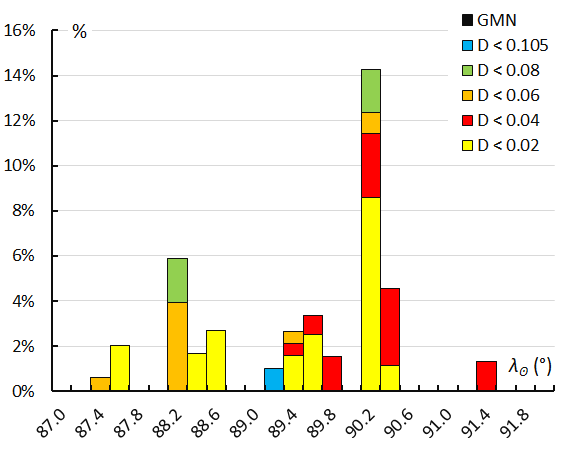
Figure 8 – The percentage of June Bootid orbits relative to the number of sporadic orbits in the a time interval of 0.2° in solar longitude for 2022 JBO-orbits with their ecliptic radiant within 96° < λ – λʘ < 110° and +54° < β < +64°.
The compactness of the main concentration in the 2022 June Bootids is obvious in the radiant plot in geocentric equatorial coordinates (Figure 9). In Sun-centered ecliptic coordinates the radiants appear less concentrated (Figure 10) and the geocentric velocity vg varies randomly within a rather small interval (Figure 11). Both diagrams of inclination i versus longitude of perihelion Π (Figure 12) and eccentricity e versus longitude of perihelion Π (Figure 13) show a very compact concentration of very similar orbits. Figure 14 shows a clear variation with higher velocities for higher inclination orbits and Figure 15 shows higher velocities for higher eccentricity.
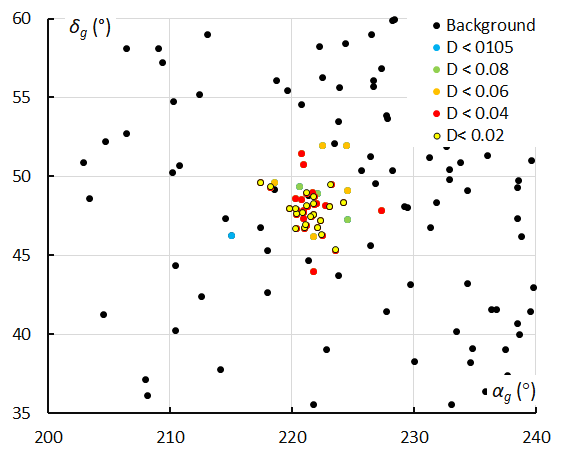
Figure 9 – The core of the 2022 June Bootid radiant in geocentric equatorial coordinates, color coded according to the similarity classes.
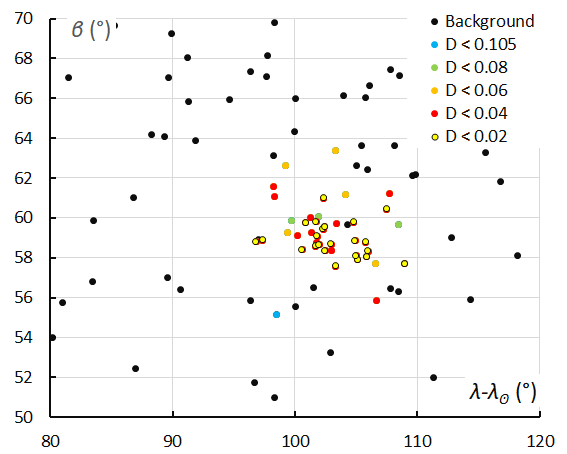
Figure 10 – The core of the 2022 June Bootid radiant in Sun-centered geocentric ecliptical coordinates, color coded according to the similarity classes.
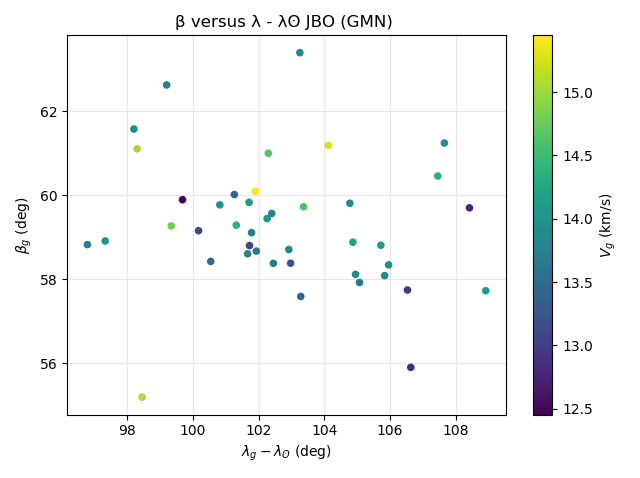
Figure 11 – The core of the 2022 June Bootid radiant in Sun-centered geocentric ecliptical coordinates, color coded according to the geocentric velocity.
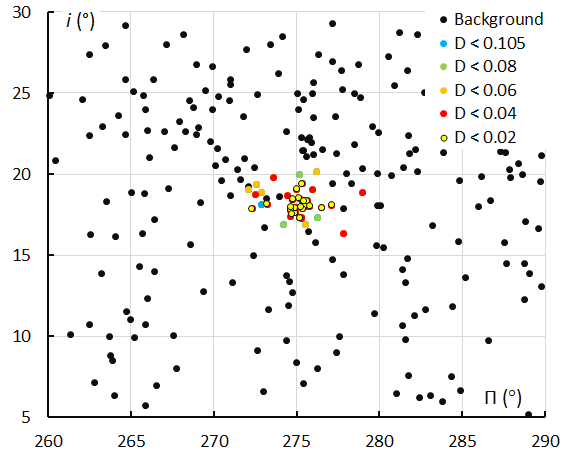
Figure 12 – Diagram of inclinination i against the longitude of perihelion Π for the core of the 2022 June Bootids, color coded according to the similarity classes.
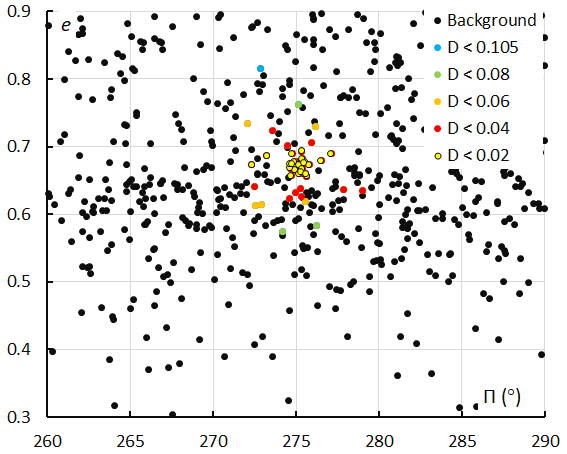
Figure 13 – Diagram of eccentricity e against the longitude of perihelion Π for the core of the 2022 June Bootids, color coded according to the similarity classes.
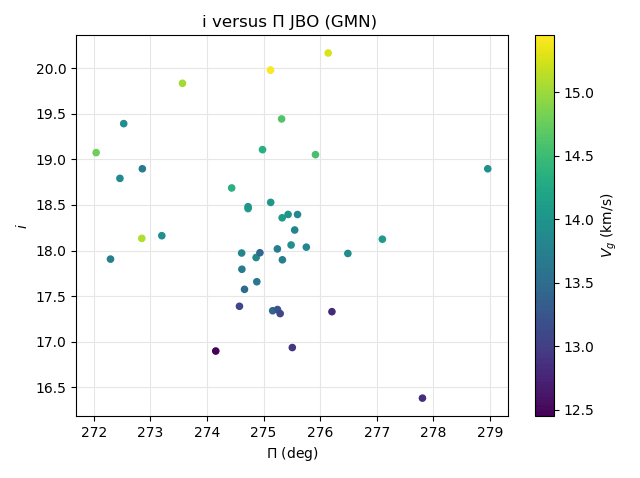
Figure 14 – Diagram of inclinination i against the longitude of perihelion Π for the core of the 2022 June Bootids, color coded according to the geocentric velocity.
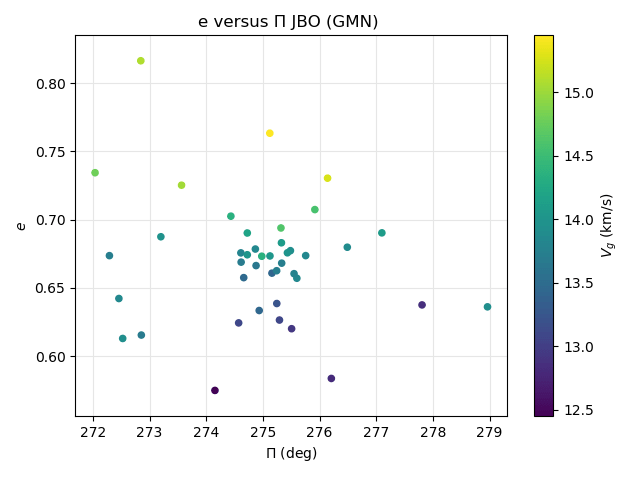
Figure 15 – Diagram of eccentricity e against the longitude of perihelion Π for the core of the 2022 June Bootids, color coded according to the similarity classes.
June Bootids in other years
EDMOND has the oldest collection of orbit data covering 2006–2016 (Kornoš et al., 2014). SonotaCo covers 2007–2021 but its data is limited to the longitudes covered from Japan (SonotaCo, 2009). CAMS made only data for 2010–2016 public (Jenniskens et al., 2011) and Global Meteor Network started collecting orbit data in October 2018.
We use our final mean orbit derived from the concentration of 23 very similar orbits (Table 2) as a reference to search the above-mentioned datasets without using any filter on the activity duration, radiant or velocity range. We find relatively many very dispersed look-alike June Bootid orbits for each dataset in each year, spread over more than two months. These can be regarded as noise or sporadics resembling the JBO-orbit. Low inclined, low velocity JFC-type meteor showers such as the June Bootids often appear problematic for identification using discrimination criteria. The results are listed in Table 3.
Because of the scatter we are only interested in the high and very high similarity classes with DSH > 0.10 and DD > 0.04 or better. Here we see mostly zero or very small numbers of orbits. EDMOND and SonotaCo had 8 orbits in 2010 that were very similar to our mean orbit. All six orbits of EDMOND occurred in the interval 92.20° < λʘ <92.33°, the two orbits of SonotaCo occurred at solar longitude 91.88° and 92.83°. In 2016 CAMS had 5 and EDMOND 2 very similar orbits. Four orbits by CAMS were recorded during the interval 89.94° < λʘ <90.17°, the last orbit at λʘ = 97.93°, the two orbits by EDMOND at solar longitude 88.92° and 89.91°. Small number statistics require caution, but the occurrence in 2010, 2016 and 2022, six years apart correspond to the periodicity of the parent body 7P/Pons-Winnecke. It is possible that some concentration of orbits, similar to the 2022 mean orbit, remained ignored in 2010 and 2016 as the camera coverage at that time was not comparable to 2022.
Table 3 – Number of orbits fulfilling the discrimination criteria with the mean orbit of 2022 as reference for the different similarity classes, for different networks in the period 2007–2022.
Year and Network | Low | Med. low | Med. high | High | Very high |
2007 SonotaCo | 8 | 2 | 0 | 0 | 0 |
2007 EDMOND | 0 | 0 | 0 | 0 | 0 |
2008 SonotaCo | 16 | 7 | 3 | 0 | 0 |
2008 EDMOND | 2 | 0 | 0 | 0 | 0 |
2009 SonotaCo | 22 | 8 | 4 | 2 | 0 |
2009 EDMOND | 4 | 4 | 1 | 0 | 0 |
2010 SonotaCo | 32 | 19 | 8 | 5 | 2 |
2010 EDMOND | 43 | 28 | 21 | 14 | 6 |
2011 SonotaCo | 13 | 3 | 1 | 0 | 0 |
2011 EDMOND | 50 | 18 | 5 | 2 | 0 |
2011 CAMS | 129 | 61 | 29 | 9 | 0 |
2012 SonotaCo | 12 | 5 | 3 | 0 | 0 |
2012 EDMOND | 49 | 18 | 4 | 0 | 0 |
2012 CAMS | 129 | 61 | 29 | 9 | 0 |
2013 SonotaCo | 18 | 7 | 1 | 0 | 0 |
2013 EDMOND | 59 | 20 | 7 | 2 | 0 |
2013 CAMS | 209 | 93 | 30 | 7 | 1 |
2014 SonotaCo | 21 | 13 | 1 | 0 | 0 |
2014 EDMOND | 80 | 30 | 6 | 2 | 0 |
2014 CAMS | 339 | 151 | 58 | 14 | 0 |
2015 SonotaCo | 19 | 12 | 2 | 0 | 0 |
2015 EDMOND | 82 | 40 | 13 | 3 | 0 |
2015 CAMS | 298 | 154 | 55 | 8 | 1 |
2016 SonotaCo | 26 | 14 | 5 | 1 | 0 |
2016 EDMOND | 101 | 49 | 20 | 7 | 2 |
2016 CAMS | 250 | 146 | 62 | 25 | 5 |
2017 SonotaCo | 35 | 14 | 5 | 0 | 0 |
2018 SonotaCo | 26 | 8 | 2 | 0 | 0 |
2019 SonotaCo | 33 | 11 | 3 | 2 | 0 |
2019 GMN | 123 | 58 | 18 | 3 | 0 |
2020 SonotaCo | 32 | 15 | 5 | 0 | 0 |
2020 GMN | 516 | 251 | 84 | 31 | 3 |
2021 SonotaCo | 28 | 12 | 4 | 1 | 0 |
2021 GMN | 507 | 241 | 101 | 27 | 0 |
2022 GMN | 2079 | 1461 | 205 | 61 | 24 |
The number of low, medium low and medium high threshold similarity orbits has been mentioned pro-memory in Table 3, as most of these are just look-alike June Bootids by pure chance. Caution is required when applying discrimination criteria, especially with this kind of JFC-type meteoroid streams.
Conclusions
The Global Meteor Network recorded a distinct concentration of June Bootid orbits with most of the orbits collected at λʘ = 90.2°, or 2022, June 21 at 22h UT. The radiant plot shows a compact core of very similar orbits embedded within a very large radiant area with very dispersed radiants with a low threshold of similarity. It is statistically impossible to distinguish the dispersed look-alike JBO-orbits from the background noise as many may be as much sporadic fitting the similarity criteria by pure chance as these could be physically related to the meteoroid stream but too dispersed to be statistically identified.
Comparing with other networks in previous years suggests that a concentration of very similar orbits like in 2022 may have occurred in 2016 and 2010. The final mean orbits for 2010, 2016 and 2022 are compared in Table 4.
Table 4 – The mean orbits for the orbits that fulfilled DD < 0.02 in 2010 (2 orbits by SonotaCo, 6 orbits by EDMOND) and 2016 (5 orbits by CAMS, 2 orbits by EDMOND), compared with the mean orbit obtained by Global Meteor Network for the June Bootids in 2022.
2010 | 2016 | 2022 | |
λʘ (°) | 92.2 | 90.1 | 89.64° |
αg (°) | 223.4 | 220.1 | 221.5° |
δg (°) | +47.3 | +48.6 | +47.9° |
vg (km/s) | 14.0 | 13.8 | 13.9 |
λ – λʘ (°) | 102.5 | 100.4 | 102.5° |
β (°) | +59.3 | +59.1 | +58.8° |
Hb (km) | 87.9 | 91.4 | 91.0 |
He (km) | 80.3 | 82.9 | 80.5 |
a (AU) | 3.11 | 3.05 | 3.11 |
q (AU) | 1.0143 | 1.0149 | 1.0141 |
e | 0.6739 | 0.6673 | 0.6739 |
ω (°) | 185.6 | 183.8 | 185.6° |
Ω (°) | 92.27 | 91.02 | 89.48° |
i (°) | 18.5 | 17.9 | 18.2° |
Π (°) | 277.8 | 274.9 | 275.1° |
Tj | 2.76 | 2.79 | 2.76 |
N | 8 | 7 | 23 |
Acknowledgment
The authors thank all the camera operators and people involved in the Global Meteor Network. Orbits for this shower analysis were obtained by GMN RMS cameras. The Global Meteor Network (GMN) data are released under the following license.
The Global Meteor Network results were obtained thanks to the efforts of the following volunteers: Richard Abraham, Victor Acciari, Rob Agar, Yohsuke Akamatsu, David Akerman, Daknam Al-Ahmadi, Jamie Allen, Alexandre Alves, Don Anderson, Željko Andreić, Martyn Andrews, Enrique Arce, Georges Attard, Chris Baddiley, David Bailey, Roger Banks, Hamish Barker, Jean-Philippe Barrilliot, Ricky Bassom, Richard Bassom, Alan Beech, Dennis Behan, Ehud Behar, Josip Belas, Alex Bell, Florent Benoit, Serge Bergeron, Denis Bergeron, Jorge Bermúdez Augusto Acosta, Steve Berry, Adrian Bigland, Chris Blake, Arie Blumenzweig, Ventsislav Bodakov, Robin Boivin, Claude Boivin, Bruno Bonicontro, Fabricio Borges, Ubiratan Borges, Dorian Božičević, David Brash, Stuart Brett, Ed Breuer, Martin Breukers, John W. Briggs, Gareth Brown, Peter G. Brown, Laurent Brunetto, Tim Burgess, Jon Bursey, Yong-Ik Byun, Ludger Börgerding, Sylvain Cadieux, Peter Campbell-Burns, Andrew Campbell-Laing, Pablo Canedo, Seppe Canonaco, Jose Carballada, Steve Carter, David Castledine, Gilton Cavallini, Brian Chapman, Jason Charles, Matt Cheselka, Enrique Chávez Garcilazo, Tim Claydon, Trevor Clifton, Manel Colldecarrera, Michael Cook, Bill Cooke, Christopher Coomber, Brendan Cooney, Jamie Cooper, Andrew Cooper, Edward Cooper, Paul Cox, Llewellyn Cupido, Christopher Curtis, Chris Dakin, Fernando Dall’Igna, James Davenport, Richard Davis, Steve Dearden, Christophe Demeautis, Bart Dessoy, Pat Devine, Miguel Diaz Angel, Paul Dickinson, Ivo Dijan, Pieter Dijkema, Tammo Dijkema Jan, Luciano Diniz Miguel, Marcelo Domingues, Stacey Downton, Stewart Doyle, Zoran Dragić, Iain Drea, Igor Duchaj, Jean-Paul Dumoulin, Garry Dymond, Jürgen Dörr, Robin Earl, Howard Edin, Ollie Eisman, Carl Elkins, Ian Enting Graham, Peter Eschman, Nigel Evans, Bob Evans, Bev M. Ewen-Smith, Seraphin Feller, Eduardo Fernandez Del Peloso, Andres Fernandez, Andrew Fiamingo, Barry Findley, Rick Fischer, Richard Fleet, Jim Fordice, Kyle Francis, Jean Francois Larouche, Patrick Franks, Stefan Frei, Gustav Frisholm, Jose Galindo Lopez, Pierre Gamache, Mark Gatehouse, Ivan Gašparić, Chris George, Megan Gialluca, Kevin Gibbs-Wragge, Marc Gilart Corretgé, Jason Gill, Philip Gladstone, Uwe Glässner, Chuck Goldsmith, Hugo González, Nikola Gotovac, Neil Graham, Pete Graham, Colin Graham, Sam Green, Bob Greschke, Daniel J. Grinkevich, Larry Groom, Dominique Guiot, Tioga Gulon, Margareta Gumilar, Peter Gural, Nikolay Gusev, Kees Habraken, Alex Haislip, John Hale, Peter Hallett, Graeme Hanigan, Erwin Harkink, Ed Harman, Marián Harnádek, Ryan Harper, David Hatton, Tim Havens, Mark Haworth, Paul Haworth, Richard Hayler, Andrew Heath, Sam Hemmelgarn, Rick Hewett, Nicholas Hill, Lee Hill, Don Hladiuk, Alex Hodge, Simon Holbeche, Jeff Holmes, Steve Homer, Matthew Howarth, Nick Howarth, Jeff Huddle, Bob Hufnagel, Roslina Hussain, Jan Hykel, Russell Jackson, Jean-Marie Jacquart, Jost Jahn, Nick James, Phil James, Ron James Jr, Rick James, Ilya Jankowsky, Alex Jeffery, Klaas Jobse, Richard Johnston, Dave Jones, Fernando Jordan, Romulo Jose, Edison José Felipe Pérezgómez Álvarez, Vladimir Jovanović, Alfredo Júnior Dal’Ava, Javor Kac, Richard Kacerek, Milan Kalina, Jonathon Kambulow, Steve Kaufman, Paul Kavanagh, Ioannis Kedros, Jürgen Ketterer, Alex Kichev, Harri Kiiskinen, Jean-Baptiste Kikwaya, Sebastian Klier, Dan Klinglesmith, John Kmetz, Zoran Knez, Korado Korlević, Stanislav Korotkiy, Danko Kočiš, Bela Kralj Szomi, Josip Krpan, Zbigniew Krzeminski, Patrik Kukić, Reinhard Kühn, Remi Lacasse, Gaétan Laflamme, Steve Lamb, Hervé Lamy, Jean Larouche Francois, Ian Lauwerys, Peter Lee, Hartmut Leiting, David Leurquin, Gareth Lloyd, Robert Longbottom, Eric Lopez, Paul Ludick, Muhammad Luqmanul Hakim Muharam, Pete Lynch, Frank Lyter, Guy Létourneau, Angélica López Olmos, Anton Macan, Jonathan Mackey, John Maclean, Igor Macuka, Nawaz Mahomed, Simon Maidment, Mirjana Malarić, Nedeljko Mandić, Alain Marin, Bob Marshall, Colin Marshall, Gavin Martin, José Martin Luis, Andrei Marukhno, José María García, Keith Maslin, Nicola Masseroni, Bob Massey, Jacques Masson, Damir Matković, Filip Matković, Dougal Matthews, Phillip Maximilian Grammerstorf Wilhelm, Michael Mazur, Sergio Mazzi, Stuart McAndrew, Lorna McCalman, Alex McConahay, Charlie McCormack, Mason McCormack, Robert McCoy, Vincent McDermott, Tommy McEwan, Mark McIntyre, Peter McKellar, Peter Meadows, Edgar Mendes Merizio, Aleksandar Merlak, Filip Mezak, Pierre-Michael Micaletti, Greg Michael, Matej Mihelčić, Simon Minnican, Wullie Mitchell, Georgi Momchilov, Dean Moore, Nelson Moreira, Kevin Morgan, Roger Morin, Nick Moskovitz, Daniela Mourão Cardozo, Dave Mowbray, Andrew Moyle, Gene Mroz, Brian Murphy, Carl Mustoe, Juan Muñoz Luis, Przemek Nagański, Jean-Louis Naudin, Damjan Nemarnik, Attila Nemes, Dave Newbury, Colin Nichols, Nick Norman, Philip Norton, Zoran Novak, Gareth Oakey, Perth Observatory Volunteer Group, Washington Oliveira, Jorge Oliveira, Jamie Olver, Christine Ord, Nigel Owen, Michael O’Connell, Dylan O’Donnell, Thiago Paes, Carl Panter, Neil Papworth, Filip Parag, Ian Parker, Gary Parker, Simon Parsons, Ian Pass, Igor Pavletić, Lovro Pavletić, Richard Payne, Pierre-Yves Pechart, Holger Pedersen, William Perkin, Enrico Pettarin, Alan Pevec, Mark Phillips, Anthony Pitt, Patrick Poitevin, Tim Polfliet, Renato Poltronieri, Derek Poulton, Janusz Powazki, Aled Powell, Alex Pratt, Miguel Preciado, Nick Primavesi, Paul Prouse, Paul Pugh, Chuck Pullen, Terry Pundiak, Lev Pustil’Nik, Dan Pye, Nick Quinn, Chris Ramsay, David Rankin, Steve Rau, Dustin Rego, Chris Reichelt, Danijel Reponj, Fernando Requena, Maciej Reszelsk, Ewan Richardson, Martin Richmond-Hardy, Mark Robbins, Martin Robinson, David Robinson, Heriton Rocha, Herve Roche, Paul Roggemans, Adriana Roggemans, Alex Roig, David Rollinson, Andre Rousseau, Jim Rowe, Nicholas Ruffier, Nick Russel, Dmitrii Rychkov, Robert Saint-Jean, Michel Saint-Laurent, Clive Sanders, Jason Sanders, Ivan Sardelić, Rob Saunders, John Savage, Lawrence Saville, Vasilii Savtchenko, Philippe Schaack, William Schauff, Ansgar Schmidt, Yfore Scott, James Scott, Geoff Scott, Jim Seargeant, Jay Shaffer, Steven Shanks, Mike Shaw, Jamie Shepherd, Angel Sierra, Ivo Silvestri, François Simard, Noah Simmonds, Ivica Skokić, Dave Smith, Ian A. Smith, Tracey Snelus, Germano Soru, Warley Souza, Mark Spink, Denis St-Gelais, James Stanley, Radim Stano, Laurie Stanton, Robert D. Steele, Yuri Stepanychev, Graham Stevens, Richard Stevenson, Thomas Stevenson, Peter Stewart, William Stewart, Paul Stewart, Con Stoitsis, Andrea Storani, Andy Stott, David Strawford, Claude Surprenant, Rajko Sušanj, Jeremy Taylor, Yakov Tchenak, John Thurmond, Stanislav Tkachenko, Eric Toops, Torcuill Torrance, Steve Trone, Wenceslao Trujillo, John Tuckett, Sofia Ulrich, Jonathan Valdez Aguilar Alexis, Edson Valencia Morales, Myron Valenta, Jean Vallieres, Paraksh Vankawala, Neville Vann, Marco Verstraaten, Arie Verveer, Jochen Vollsted, Predrag Vukovic, Aden Walker, Martin Walker, Bill Wallace, John Waller, Jacques Walliang, Didier Walliang, Christian Wanlin, Tom Warner, Neil Waters, Steve Welch, Tobias Westphal, Tosh White, Alexander Wiedekind-Klein, John Wildridge, Ian Williams, Mark Williams, Guy Williamson, Graham Winstanley, Urs Wirthmueller, Bill Witte, Jeff Wood, Martin Woodward, Jonathan Wyatt, Anton Yanishevskiy, Penko Yordanov, Stephane Zanoni, Pető Zsolt, Dario Zubović, Marcelo Zurita, Rob de Corday Long, Pierre de Ponthière, Jocimar Justino de Souza, Erwin van Ballegoij, Raoul van Eijndhoven, Ivica Ćiković, Dino Čaljkušić, Damir Šegon, Marko Šegon (list established 19 December 2022).
References
Denning W. F. (1916). “Remarkable Meteoric Shower on June 28”. Monthly Notices of the Royal Astronomical Society, 76, 740–743.
Drummond J. D. (1981). “A test of comet and meteor shower associations”. Icarus, 45, 545–553.
Jenniskens P. (2006). Meteor Showers and their Parent Comets. ISBN 0521853494. Cambridge, UK: Cambridge University Press. Pages 528–530.
Jenniskens P., Gural P. S., Grigsby B., Dynneson L., Koop M. and Holman D. (2011). “CAMS: Cameras for Allsky Meteor Surveillance to validate minor meteor showers”. Icarus, 216, 40–61.
Jenniskens P., Baggaley J., Crumpton I., Aldous P., Pokorny P., Janches D., Gural P. S., Samuels D., Albers J., Howell A., Johannink C., Breukers M., Odeh M., Moskovitz N., Collison J. and Ganjuag S. (2018). “A survey of southern hemisphere meteor showers”. Planetary Space Science, 154, 21–29.
Jopek T. J. (1993). “Remarks on the meteor orbital similarity D-criterion”. Icarus, 106, 603–607.
Jopek T. J., Rudawska R. and Pretka-Ziomek H. (2006). “Calculation of the mean orbit of a meteoroid stream”. Monthly Notices of the Royal Astronomical Society, 371, 1367–1372.
King A. (1927). “Meteor notes. – The Pons-winneckids 1927”. The Observatory, 50, 361–363.
Kornoš L., Matlovič P., Rudawska R., Tóth J., Hajduková M. Jr., Koukal J. and Piffl R. (2014). “Confirmation and characterization of IAU temporary meteor showers in EDMOND database”. In Jopek T. J., Rietmeijer F. J. M., Watanabe J., Williams I. P., editors, Proceedings of the Meteoroids 2013 Conference, Poznań, Poland, Aug. 26-30, 2013. A.M. University, pages 225–233.
Moorhead A., Clements T.D. and Vida D. (2020). “Realistic gravitational focusing of meteoroid streams”. Monthly Notices of the Royal Astronomical Society, 494, 2982–2994.
Roggemans P., Johannink C. and Cambell-Burns P. (2019). “October Ursae Majorids (OCU#333)”. eMetN, 4, 55–64.
SonotaCo (2009). “A meteor shower catalog based on video observations in 2007–2008”. WGN, Journal of the IMO, 37, 55–62.
Southworth R. B. and Hawkins G. S. (1963). “Statistics of meteor streams”. Smithsonian Contributions to Astrophysics, 7, 261–285.
Spurny P., Borovicka J. (1998). “June Bootid meteors 1998”. Central Bureau for Astronomical Telegrams, Circular No. 6973.
Velkov V. (1999). “The 1998 June Bootid Outburst Observed in Bulgaria”. In, Arlt R., Knöfel A., editors, Proceedings of the International Meteor Conference, Stará Lesná 20-23 August 1998. International Meteor Organization, Pages 84–96.
Vida D., Gural P., Brown P., Campbell-Brown M., Wiegert P. (2019). “Estimating trajectories of meteors: an observational Monte Carlo approach – I. Theory”. Monthly Notices of the Royal Astronomical Society, 491, 2688–2705.
Vida D., Gural P., Brown P., Campbell-Brown M., Wiegert P. (2020). “Estimating trajectories of meteors: an observational Monte Carlo approach – II. Results”. Monthly Notices of the Royal Astronomical Society, 491, 3996–4011.
Vida D., Šegon D., Gural P. S., Brown P. G., McIntyre M. J., Eschman P., Roggemans P., Merlak A., Zubrović D. (2021). “The Global Meteor Network – Methodology and first results”. Monthly Notices of the Royal Astronomical Society, 506, 5046–5074.
Yamamoto I. (1922). “Observations in Japan of meteors probably connected with Pons Winnecke’s Comet”. The Observatory, 45, 81–83.